Unveiling the Enigma: Deciphering the Secrets of Open-Shell Catalysts
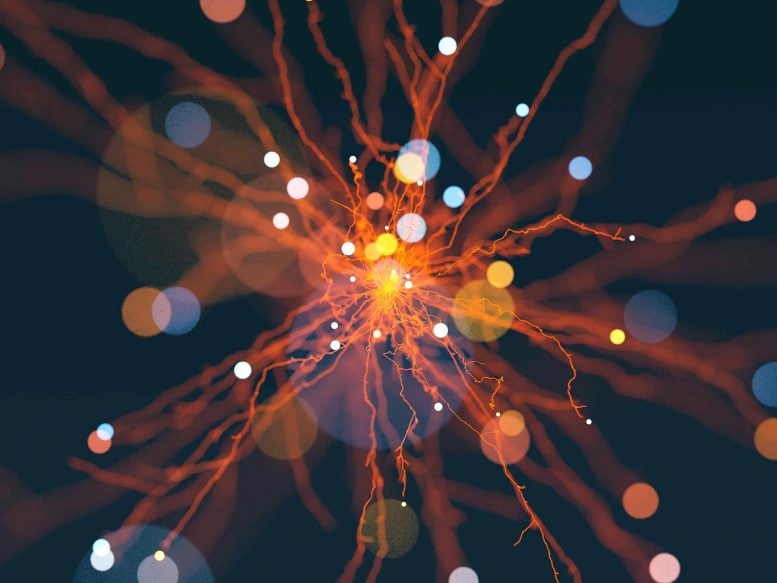
A new study reveals the role of spin effects on iron-catalyzed hydrosilylation and their impact on catalytic behavior, accelerating reaction rates and enhancing regioselectivity. This could have significant implications for catalyst design advancements.
There are two primary types of metal complex catalysts based on their spin states: closed-shell and open-shell catalysts. The former lack unpaired electrons and are typically based on noble metals such as palladium. They have been extensively studied and are widely used in industry. Open-shell catalysts, on the other hand, have unpaired electrons and are often derived from more common metals like iron. They represent a different approach.
These open-shell catalysts travel different potential energy surfaces via spin transitions, displaying catalytic behaviors noticeably different from their closed-shell counterparts. This difference opens up exciting new possibilities in synthetic chemistry and is attracting growing interest.
Despite this, the development of open-shell catalysts is slowed by a limited knowledge of their spin effects and the lack of effective control methods. Understanding these spin effects is critical to enhancing the design of readily available metal catalysts and could potentially revolutionize catalysis, an area of considerable research importance.
Iron-catalyzed hydrosilylation of alkynes involves two potential energy surfaces, the triplet (red) and quintet (blue) states, where the spin crossover effectively reduces the reaction energy barrier, while the spin-delocalization between iron and ligand dynamically controls the oxidation and spin states of the metal center.
To address these scientific hurdles, Shou-Fei Zhu's team at Nankai University performed an extensive study on the spin effects in iron-catalyzed hydrosilylation of alkynes, combining experimental work with theoretical computations. They discovered a unique mechanism by which the spin state of the open-shell iron catalyst modulates both reactivity and selectivity. Peng He, a doctoral student at Nankai University, is the first author of these findings recently published online in the National Science Review.
The team synthesized a range of active iron complexes, and established their structures through X-ray single-crystal diffraction. They analyzed the magnetic properties, metal valence states, and spin multiplicity of the iron center using methods including superconducting quantum interferometry, X-ray photoelectron spectroscopy, and Mössbauer spectroscopy.
Theoretical calculations exposed the critical role of spin-delocalization interactions between iron and the 1,10-phenanthroline ligand in controlling the iron center's spin and oxidation states. This regulation forms the structural basis for the unique spin effects observed in iron catalysts.
Controlled experiments highlighted that the reaction goes as a two-electron redox process, promoted by zero-valent iron species. These stages happen on potential energy surfaces of different spin multiplicities, with the iron catalyst enabling transitions between these surfaces through spin crossover. This adaptability fulfills the contrasting electrostatic demands of oxidative addition and reductive elimination, substantially reducing the energy barriers of these elementary processes, thereby boosting the reaction rate.
Spin effects also considerably impact high regioselectivity. Iron catalysts adjust the spin delocalization states of complexes through specific spin states. These adjustments manipulate the intramolecular noncovalent interactions within transition states, impacting their stability and allowing precise control of regioselectivity.
In conclusion, this research brings new understanding to the spin effect in iron-catalyzed hydrosilylation of alkynes. The catalyst dynamically manages the iron center's spin and oxidation states through spin-delocalization, supporting both oxidative addition and reductive elimination processes with opposing electrostatic requirements in the catalytic cycle. Additionally, it influences regioselectivity by changing noncovalent interactions in the transition states. These discoveries could guide the exploration and application of open-shell catalysts.