Uncovering the Molecular Mechanisms of Hibernation in Mammals
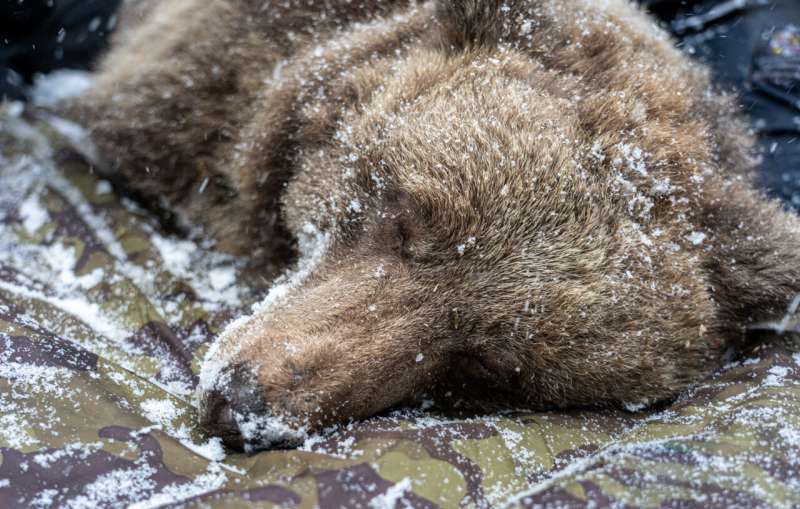
February 20, 2024
This article has gained approval via Science X's editorial procedures and standards. The editors have assessed these main features to confirm the authenticity of the content:
- Fact verification
- Validated sources
- Editing for clarity
Endorsed by eLife
Today, scientists unveiled their research on the molecular machinations behind hibernation in a reviewed preprint published in eLife.
The study was carried out using both small and large hibernating mammals. The editors recognized it for advancing our understanding of how myosin structure and energy use influence the molecular facets of hibernation. The study relied on robust methodology and concrete evidence. Results suggest that myosin, a motor protein necessary for muscle contraction, may play a part in non-shivering thermogenesis - heat production independent of the muscular action of shivering - during hibernation.
Hibernation is a widespread survival tactic among animals, characterized by deep sleep, significant reductions in metabolism, body temperature, heart rate, and respiration. During hibernation, animals utilize stored energy reserves, primarily fats, to maintain bodily functions. This metabolic slowdown lets hibernating animals save energy, tolerating prolonged periods of food shortage and severe winter conditions. The underlying cellular and molecular mechanisms of hibernation, however, remain only partially understood.
Smaller hibernating mammals undergo long episodes of a hypometabolic condition known as torpor. This state significantly reduces their body temperature, interrupted by spontaneous periods of interbout euthermic arousals (IBA), during which they temporarily increase their body temperature to resume some physiological functions like elimination of waste and additional food consumption.
In contrast, larger mammals have a less drastic reduction in body temperature during hibernation, maintaining it reasonably steady. Skeletal muscles, comprising around half of a mammal's body mass, play a crucial role in determining their heat generation and energy expenditure.
'Until recently, we thought energy use in skeletal muscles was strongly tied to the activity of myosin, which contributes to muscle contraction. But there's emerging evidence suggesting that even when relaxed, skeletal muscles still use a small amount of energy,' says lead author, Christopher Lewis, a postdoctoral researcher from the Department of Biomedical Sciences at the University of Copenhagen, Denmark.
'In resting muscles, myosin heads can occupy different states: the 'disordered-relaxed,' or DRX state, and the 'super-relaxed,' or SRX state. Myosin heads in DRX consume ATP, cells' energy currency, five to ten times faster than in the SRX state,' Lewis further provides.
Believing that fluctuations in the proportion of myosin in the DRX or SRX states might account for the decreased energy use observed during hibernation, Lewis and team tested this hypothesis. They extracted skeletal muscle samples from two small hibernators, the ground squirrel and garden dormouse, and two large ones, the American black bear and brown bear.
The team first sought to determine whether there were any differences in myosin states, and their corresponding ATP consumption rates, between periods of activity and hibernation. They examined muscle fibers taken from the two bear species during their active summer phase (SA), and their winter hibernation period.
They found no differences in the proportion of myosin in the DRX or SRX state between the two phases. To assess the rate of ATP consumption by myosin, they used a special test known as the Mant-ATP chase assay. This revealed that energy consumption rates of myosin didn't change either. This could potentially prevent significant muscle wasting in bears during hibernation.
The researchers also conducted the Mant-ATP chase assay on samples taken from the small mammals during SA, IBA and torpor periods. Similar to the larger hibernators, they also didn't observe any differences in the proportion of myosin heads in the SRX or DRX state between the three phases. However, they discovered that the ATP turnover time of myosin molecules in both states was lower in IBA and torpor compared to SA phase, leading to an unexpected increase in ATP consumption.
As body temperature of small mammals drops more dramatically during hibernation than that of larger ones, the team examined whether this unanticipated increase in ATP use also happened at lower temperatures. They repeated the Mant-ATP chase assay at a temperature of 8°C, instead of the previous lab temperature of 20°C. Reducing the temperature resulted in decreased DRX and SRX-linked ATP turnover times in SA and IBA, suggesting an increase in ATP consumption.
Metabolic organs, such as skeletal muscle, are well known to increase core body temperature in response to significant cold exposure, either by inducing shivering or through non-shivering thermogenesis. Cold exposure caused an increase in ATP consumption by myosin in samples obtained during SA and IBA, suggesting that myosin may contribute to non-shivering thermogenesis in small hibernators.
The team did not observe cold-induced changes in myosin energy consumption in samples obtained during torpor. They suggest that this is likely a protective mechanism to maintain the low core body temperature, and wider metabolic shutdown, seen during torpor.
Finally, the researchers wanted to understand the changes that occur at the protein level during the different hibernating phases. They assessed whether hibernation affects the structure of two myosin proteins from the thirteen-lined ground squirrel, Myh7 and Myh2. Although they did not observe any hibernation-related changes in the structure of Myh7, they discovered that Myh2 underwent significant phosphorylation—a process crucial for energy storage—during torpor, compared to SA and IBA.
They also analyzed the structure of the two proteins in the brown bear, finding no structural differences between SA and hibernation. They therefore conclude that Myh2 hyper-phosphorylation is specifically associated with torpor, rather than hibernation in general, and propose that this serves to increase myosin stability in small mammals. This may act as a potential molecular mechanism to mitigate myosin-associated increases in skeletal muscle expenditure in response to cold exposure during periods of torpor.
eLife's editors note that some areas of the study warrant further study. Namely, the muscle samples were taken exclusively from the legs of the animals studied. Given the core body and limbs have different temperatures, investigating muscle samples from other areas of the body would further validate the team's findings.
'Altogether, our findings suggest that ATP turnover adaptations in DRX and SRX myosin states occur in small mammals like the thirteen-lined ground squirrel during hibernation in cold environments. In contrast, larger mammals like the American black bear show no such changes, likely due to their stable body temperature during hibernation,' concludes senior author Julien Ochala, Associate Professor at the Department of Biomedical Sciences, University of Copenhagen. 'Our results also suggest that myosin may act as a contributor to skeletal muscle non-shivering thermogenesis during hibernation.'
Provided by eLife