Cracking the Mystery of Iron-60: Decrypting Celestial Explosions on our Planet
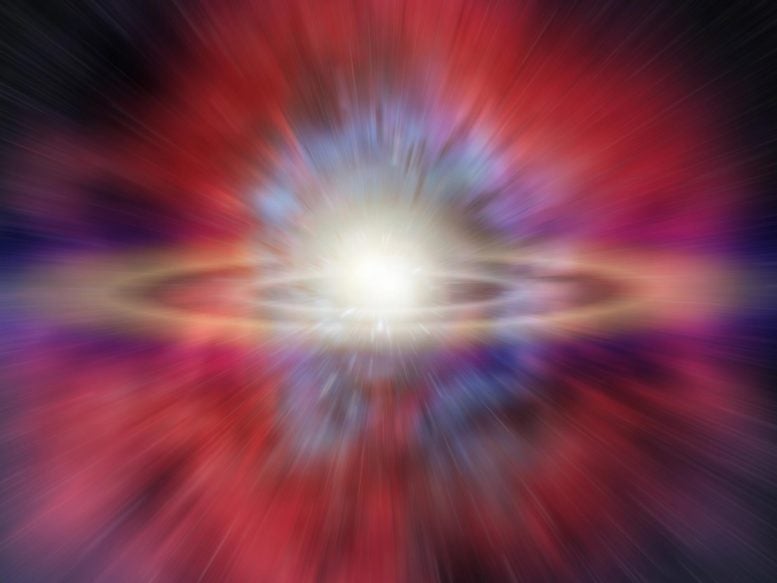
The marks of close supernovae are imprinted on Earth in the form of unique isotopes such as iron-60 and plutonium-244, identifiable in lunar samples and sediments. These cosmic by-products have been distinguished with the help of progressive methods like accelerator mass spectrometry, giving us profound insights into the occurrence of massive stellar explosions in our cosmic vicinity.
When substantial stars or celestial entities explode near the Earth, the rubble can reach our solar system. Proof of these cosmic incidents are available both on Earth and the Moon, and can be identified via accelerator mass spectrometry (AMS). Prof. Anton Wallner of the Helmholtz-Zentrum Dresden-Rossendorf (HZDR) recently provided a comprehensive review of this intriguing research in the publication, Annual Review of Nuclear and Particle Science. He is set to considerably push this promising field further with the ultra-sensitive AMS facility 'HAMSTER'.
In their published study, HZDR physicist Anton Wallner and partner Prof. Brian D. Fields from the University of Illinois in Urbana, USA, provide an extensive image of near-earth cosmic explosions with a specific emphasis on events that took place three million and seven million years ago, respectively.
"Luckily, these incidents were distanced enough that they probably had no significant influence on the Earth's climate or any substantial effects on the biosphere. However, if cosmic explosions occur within 30 light-years, it could lead to serious complications," Wallner explains. When translated into parsec, an astronomical unit, this equates to less than eight to ten parsecs.
Once large stars have exhausted their fuel, their cores implode into an ultra-dense neutron star or a black hole, while simultaneously, scorching gas is thrust outward at a high pace. An expanding shock wave carries a significant fraction of the gas and dust dispersed between the stars. This envelope, similar to an immense balloon with irregularities, also encompasses any material already present in space. After several thousand years, supernova remnants expand to several tens of parsecs in diameter, continuously slowing their spread until they finally come to a halt.
AMS measurements of a ferromanganese crust from the Pacific Ocean discovered interstellar iron-60, manganese-53, and plutonium-244. These atoms are dated over a timeline of more than 20 million years. As a scale, the coin has a diameter of 3.2 cm. Credit: HZDR.
Nearby cosmic explosions possess the potential to seriously interrupt Earth's biosphere, leading to mass extinction similar to an asteroid impact 66 million years ago. That event led to the extinction of many species, including dinosaurs. "Considering the timescale since the formation of our solar system, spanning billions of years, it cannot be ruled out that very close cosmic explosions have happened," Wallner stresses.
However, supernovae happen only in incredibly massive stars, those with a mass eight to ten times that of our Sun. These types of stars are rare. Red supergiant Betelgeuse in the Orion constellation, roughly 150 parsecs away from our solar system, is one of the closest stars of this size.
A large number of new atoms is produced just before and during a supernova or other cosmic explosions. Wallner has a keen interest in the radioactive isotope of iron with an atomic mass of 60. About half of these isotopes, known as iron-60, have transformed into a stable nickel isotope after 2.6 million years. Hence, all the iron-60 existing at the Earth's formation around 4,500 million years ago has since vanished.
“Iron-60 is extremely scarce on Earth as it's not produced in any substantial quantity through natural means. However, it is generated in abundance just prior to a supernova explosion. If this isotope now appears in sediments from the ocean floor or in the material from the Moon's surface, it has likely come from a supernova or a similar process in space that occurred near the Earth just a few million years ago,” Wallner concludes.
Prof. Anton Wallner, a HZDR physicist, is an expert in searching for interstellar matter using Accelerator Mass Spectrometry (AMS). Currently, Wallner and his colleagues from Australia are hunting for additional cosmic isotopes – in Canberra, he is searching for Fe-60 atoms, in Sydney for PU-244 atoms. He is conducting this research with a collection of lunar samples received from the US space agency NASA. Credit: ANU.
The principle remains the same for the plutonium isotope with an atomic mass of 244. However, it’s more likely that this plutonium-244 is formed through the collision of neutron stars rather than from supernovae. As such, it serves as a marker for the nucleosynthesis of heavy elements. After about 80 million years, roughly half of the plutonium-244 isotope transforms into different elements. So, alongside iron-60, the sluggishly decaying plutonium-244 is another signifier of galactic events and the synthesis of new elements over the past millions of years.
"The frequency, location, and conditions under which these heavy elements are created are subjects of active scientific discussions. Plutonium-244 also needs explosive incidents and theoretically, it’s produced in a similar way to elements like gold or platinum, which occur naturally on Earth, but today consist of stable atoms," explains Wallner.
But the critical question is, how do these isotopes end up on Earth? The atoms of iron-60, expelled by the supernova, tend to gather in dust particles, and so do the plutonium-244 isotopes, which might have been formed in other events and then gathered up by the supernova’s expanding outer region. Post cosmic explosions that occur at a distance of more than ten but less than 150 parsecs, according to the theory, the solar wind and the magnetic field of the heliosphere stop individual atoms from reaching the Earth. However, the iron-60 and plutonium-244 atoms trapped in dust particles continue their journey towards the Earth and the Moon, eventually finding their way to the surface.
Even when a supernova happens within the 'kill radius' of less than ten parsecs, not even a microgram of matter from the envelope will land on each square centimeter. In fact, just a handful of iron-60 atoms per square centimeter reach the Earth each year. This presents a massive challenge for researchers like physicist Anton Wallner: Within a one-gram sediment sample, perhaps a few thousand iron-60 atoms are distributed like needles in a haystack among billions of billions of constant and stable iron atoms with the atomic mass of 56. Moreover, even the most sensitive measurement method may only detect every five thousandth particle, i.e., a maximum of just a few iron-60 atoms in a typical measurement sample.
Such incredibly low concentrations can only be identified with Accelerator Mass Spectrometry, or AMS for short. One such facility, the Dresden AMS (DREAMS), is located at the HZDR, and will soon be accompanied by the Helmholtz Accelerator Mass Spectrometer Tracing Environmental Radionuclides (HAMSTER). AMS facilities globally have been designed differently; hence, the various facilities can complement each other in the search for rare isotopes from supernova explosions.
Isotopes of the same element, but with different mass, like the naturally occurring iron-56, are removed with mass filters. Atoms of other elements with the same mass as the target iron-60, for example, the naturally present nickel-60, also cause interference. Despite very complex chemical preparation, these atoms are still billions of times more prevalent than iron-60 and have to be separated in a particular accelerator facility using nuclear physics techniques.
At the end of a several hours long measuring process, perhaps only around five individual iron-60 atoms are identified. The foundational work in iron-60 detection was done at TU Munich. Currently, however, Canberra at the Australian National University has the only facility in the world that is sensitive enough to perform such measurements.
In all, about one thousand iron-60 atoms have been measured in the past 20 years. As for the interstellar plutonium-244, which occurs in concentrations more than 10,000 times lower, individual atoms data was available for a long time. Only recently, it has been possible to identify about a hundred plutonium-244 atoms at a specialized infrastructure in Sydney – which is similar to the HAMSTER facility that's currently being developed at the HZDR.
However, only some samples are suitable for exploration as these act as archives, preserving these atoms coming from space for millions of years. Samples from the Earth's surface, for example, are rapidly "diluted" due to geological processes. Ideal samples are sediments and crusts from the deep sea, which form slowly and undisturbed on the ocean floor. Alternatively, lunar surface samples are also suitable as disruptive processes are hardly an issue there.
Until the start of November 2023, Wallner and his team will undertake a research trip to hunt for more cosmic isotopes at suitable AMS facilities in the Australian cities of Canberra (for iron-60) and Sydney (for plutonium-244). For this mission, he has received a number of lunar samples from the U.S. space agency NASA.
“Parallel measurements are also taking place at HZDR. These unique samples will allow us to gain new insights into supernova explosions near Earth, but also into the heaviest elements in our galaxy which are formed through these and other processes,” Wallner is certain.