Impact of Super Strong Magnetic Fields on Nuclear Matter
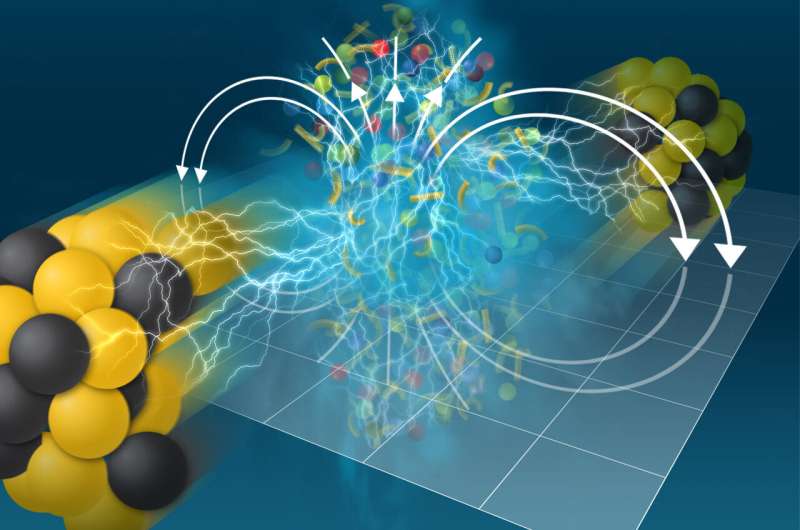
23rd February, 2024
The Science X editorial team has meticulously reviewed this article. Its credibility was ensured by incorporating the following essential elements:
Fact checking
Citation from trustworthy sources
Proofread by Karen McNulty Walsh, Peter Genzer, and Brookhaven National Laboratory
An analytical work by the STAR team at the Relativistic Heavy Ion Collider (RHIC), a particle collider operated by the U.S. Department of Energy's (DOE) Brookhaven National Laboratory, has unearthed the first direct proof of a mark abdicated by what could potentially be the most potent magnetic fields in the universe on 'deconfined' nuclear matter. The findings were deduced by measuring the separation pattern of particles with different charges following nuclear collision in this DOE Office of Science user facility.
Published in the Physical Review X journal, the data suggests the robust magnetic fields produced in askew collisions instigate an electric flow in the quarks and gluons released, or deconfined, from protons and neutrons during the particle collisions.
The new insights offer scientists a novel method to explore the electrical conductivity of this 'quark-gluon plasma' (QGP), and acquire more knowledge on these elementary constituents of atomic nuclei.
'We managed to measure, for the first time, the interplay between the magnetic field and the quark-gluon plasma (QGP),' stated Diyu Shen, a STAR physicist, and Fudan University scholar in China, who was one of the leaders of this analysis. Indeed, measuring this interaction's impact provided concrete proof of these powerful magnetic fields' existence.
Scientists have long theorized that askew collisions of heavy atomic nuclei such as gold, or heavy ions, would spawn potent magnetic fields. This is due to some of the non-colliding positively-charged protons and neutral neutrons-in the nuclei that start to rotate as the ions graze against each other at nearly the speed of light.
'It is anticipated that these rapidly-moving positive charges will create a robust magnetic field, estimated to be 1018 gauss,' explained Gang Wang, a STAR physicist from the University of California, Los Angeles. This field's strength dwarfs those of neutron stars (1014 gauss), fridge magnets (around 100 gauss), and even the Earth's protective magnetic field (0.5 gauss).
This could very well be the mightiest magnetic field in our universe.'
However, due to the rapid unfolding of events in heavy ion collisions, these fields are short-lived, decaying in less than 10-23 seconds, making them rather elusive.
Rather than attempting to measure the field directly, the STAR scientists sought to identify the magnetic field's impact on the particles that are propelled out from the collisions.
We were primarily focused on the collective movement of charged particles,' explained Wang.
Detection of deflection
Magnetic fields are known for their influence on the movement of charged particles and their ability to introduce electromagnetic fields in conductive types of matter. This logic was also applied here, albeit on a much smaller scale.
'We aimed to discern whether the charged particles produced in askew heavy ion collisions were being deviated in a manner that only an electromagnetic field in the microscopic blobs of QGP formed in these collisions could explain,' conveyed Aihong Tang, a Brookhaven Lab physicist and a member of the STAR collaboration.
The group utilized STAR's advanced detector systems to monitor the collective motion of different pairs of charged particles while eliminating the effects of unrelated non-electromagnetic interferences. The researchers were keen to discount any deflections induced by charged quarks carried along with the colliding nuclei. Luckily, the 'transported quarks' pattern of deflection is contrary to that triggered by the magnetic-field-induced electric current, also known as Faraday induction.
Eventually, we noticed a pattern of charge-dependent deflection that could only be initiated by the presence of an electromagnetic field in the QGP, a clear indication of Faraday induction,' shared Tang.
This strong signal wasn't just noticeable in off-center collisions of two gold nuclei at high energy (ie gold-gold at 200 billion electron volts, or GeV) but also in off-center collisions of smaller nuclei, like ruthenium-ruthenium and zirconium-zirconium, both at 200 GeV.
'The occurrence of this phenomenon is universal. It's not only confined to larger systems but also discernible in smaller systems,' concluded Shen.
The scientists saw an even stronger signal when they analyzed data from gold-gold collisions at a relatively low energy: 27 GeV. This finding provides more supporting evidence that the powerful magnetic fields generated by off-center collisions induced the particle-deflecting electromagnetic field.
That's because Faraday induction occurs as the magnetic field dissipates. In lower-energy collisions, that happens more slowly.
'This effect is stronger at lower energy because the lifetime of the magnetic field is longer at lower energy; the speed of the nuclear fragments is lower, so the magnetic field and its effects last longer,' said Wang.
Implications
Now that the scientists have evidence that magnetic fields induce an electromagnetic field in the QGP, they can use the induction to probe the QGP's conductivity.
'This is a fundamental and important property,' said Shen. 'We can infer the value of the conductivity from our measurement of the collective motion. The extent to which the particles are deflected relates directly to the strength of the electromagnetic field and the conductivity in the QGP—and no one has measured the conductivity of QGP before.'
Understanding the fundamental electromagnetic properties of the QGP could offer insights into important questions in physics. For one thing, the magnetic fields that induce the electromagnetic effects may contribute to an interesting separation of particles according to their 'handedness,' or chirality.
'This study gives strong evidence of the magnetic field, which is one of the preconditions for this 'chiral magnetic effect',' Shen said.
The magnetic field and electromagnetic properties of the QGP also play a role in determining the conditions under which free, deconfined quarks and gluons coalesce to form composite particles called hadrons—such as the protons and neutrons that make up ordinary nuclei.
'We want to map out the nuclear 'phase diagram,' which shows at which temperature the quarks and gluons can be considered free and at which temperature they will 'freeze out' to become hadrons. Those properties and the fundamental interactions of quarks and gluons, which are mediated by the strong force, will be modified under an extreme electromagnetic field,' said Wang.
With this new probe of the QGP's electromagnetic properties, he added, 'we can investigate these fundamental properties in another dimension to provide more information about the strong interaction.'
For now, the scientists pointed out, theorists will be looking at these results to help refine the interpretations.
Provided by Brookhaven National Laboratory