Atomic Quantum Memory Enables Synchronization of Single Photons, Say Researchers
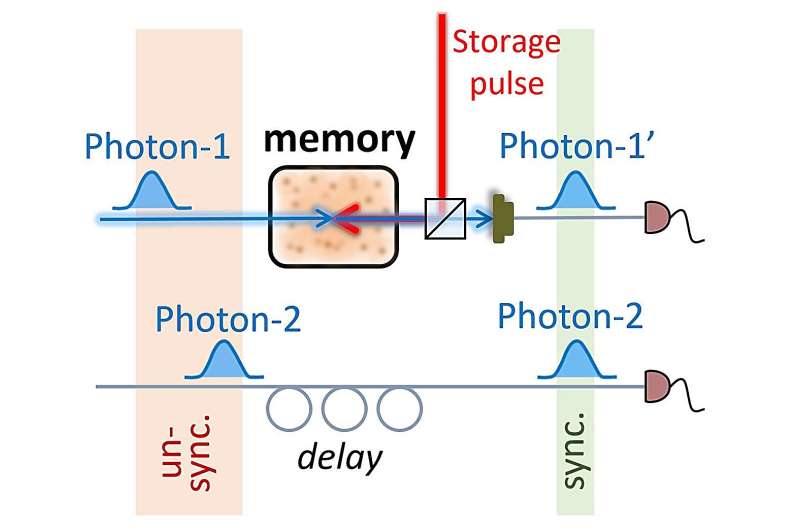
July 30, 2023
feature
This article has been reviewed according to Science X's editorial process and policies. Editors have highlighted the following attributes while ensuring the content's credibility:
- fact-checked
- peer-reviewed publication
- trusted source
- proofread
by Ingrid Fadelli Phys.org
A long-standing challenge in the field of quantum physics is the efficient synchronization of individual and independently generated photons (i.e., light particles). Realizing this would have crucial implications for quantum information processing that relies on interactions between multiple photons.
Researchers at Weizmann Institute of Science recently demonstrated the synchronization of single, independently generated photons using an atomic quantum memory operating at room-temperature. Their paper, published in Physical Review Letters, could open new avenues for the study of multi-photon states and their use in quantum information processing.
'The project idea came about several years ago, when our group and the group of Ian Walmsley demonstrated an atomic quantum memory with an inverted atomic-level scheme compared to the typical memories—the ladder memory, named fast ladder memory (FLAME),' Omri Davidson, one of the researchers who carried out the study, told Phys.org. 'These memories are fast and noise-free, and therefore they are useful for synchronization of single photons.'
Photonic quantum computation and other quantum information protocols rely on the successful generation of multi-photon states. As most quantum sources employed in research to date are probabilistic, they are unsuitable for generating multi-photon states at a reasonable rate.
As part of their recent study, Davidson and his colleagues set out to explore the possibility of realizing these states using an atomic quantum memory, devices that can store the quantum states of photons while retaining the quantum information they carry. Their prediction was that their atomic quantum memory would be able to store probabilistically generated photons and release them on-demand to generate a multi-photon state.
'The objective of the current research was to demonstrate synchronization of single photons using an independent room-temperature atomic quantum memory for the first time,' Davidson said. 'To achieve this, we had to rebuild the memory with several improvements, as well as to build a single-photon source that generates photons that can efficiently interface with the memory. Finally, we were set to demonstrate the actual photon synchronization, which interfaced the photon source and memory modules, with suitable control electronics of the experiment.'
FLAME, the quantum memory used by the researchers and developed as part of their previous research, relies on an inverted atomic-level scheme, referred to as a ladder scheme. Compared to conventional ground-state memories, which are typically slow and vulnerable to noise, FLAME is both fast and noise free, yet it can only store information for shorter periods of time. As speed and lack of noise are essential properties for the synchronization of single photons, their hope was that it would allow them to generate multi-photon quantum states.
'The second advantage of our specific ladder scheme in rubidium atoms, is the small wavelength mismatch of the signal and control light-fields transitions,' Davidson explained. 'This enables a relatively long memory lifetime compared to other ladder schemes with a larger wavelength mismatch, due to the smaller two-photon Doppler broadening. Finally, we generated the photons using the same atomic-level structure as our memory, which enables efficient coupling of the photons with the memory.'
The many advantages of the team's FLAME memory scheme collectively contributed to the success of their experiment, allowing them to synchronize individual photons at a high rate. Using their atomic quantum memory, they were able to store and retrieve single photons with an end-to-end efficiency of ηe2e=25% and final antibunching of g(2)h=0.023, attaining a rate of more than 1,000 synchronized photon pairs per second.
G (2) h, or photon antibunching, is a measure of how 'single' the single photons are. Perfect single photons have g(2)h= 0 , whereas classical light has g(2)h= 1. Thus, at g(2)h= 0.023, the photons synchronized by the researchers remain almost perfect single-photons, thanks to the noise-free operation of the memory.
'We were able to synchronize photons that are compatible with atomic systems at high rate,' Davidson said. 'Photons that are compatible with atoms are important for many photonic quantum information protocols, such as a deterministic two-qubit entangling gate. Previous photon synchronization demonstrations either used broadband photons which are not compatible with atomic systems, or photons that are compatible with atomic systems with extremely low rates.'
The photon synchronization rate that Davidson and his colleagues attained in their experiments is more than 1,000 times better than previous demonstrations using photons that are compatible with atomic systems. Their work opens new avenues for the study of interactions between multi-photon states and atoms, such as so-called deterministic two-photon entangling gates. In the future, it could have valuable implications both for the realization of quantum information processing and quantum optics systems.
'We are currently exploring two research paths,' Davidson added. 'The first is to achieve strong photon-photon interactions with rubidium atoms, in a similar system to the one used for synchronization. Achieving this goal will enable us to demonstrate a deterministic entangling gate between the synchronized single-photons.
'These gates are an important component in photonic quantum computation, as they enable to reduce the resource overhead over currently pursued methods (called linear-optics quantum computation). To date, these gates were only demonstrated with cold atoms setups, and not hot atoms, which limits the scalability of these systems.'
In their next studies, Davidson and his colleagues also plan to further develop their FLAME memory, allowing it to store a photonic qubit (i.e., a photon in a quantum superposition of two polarization states), rather than only storing individual photons in one polarization state. This could ultimately allow them to perform quantum computations using photons.
© 2023 Science X Network