Removing Aqueous Microfoulants by Microtexturing Soft Materials
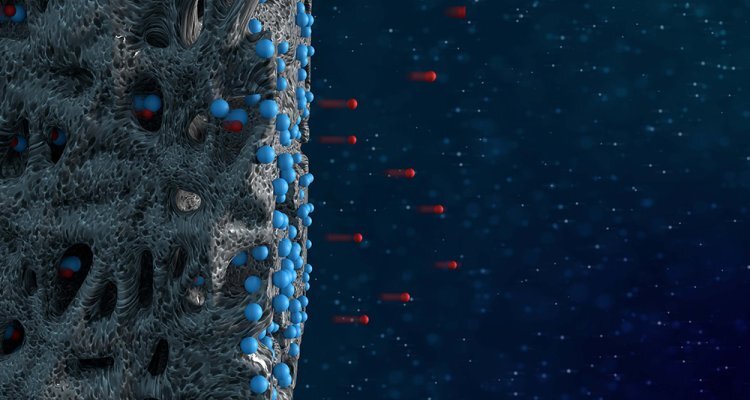
The date of publication for this article is January 5, 2024.
Under the scrutiny of Science X's editorial process and policies, this article has undergone a rigorous review. Evaluations considered factors such as fact-checking, peer-reviewed sources, and proofreading, among others.
Author Thamarasee Jeewandara and Phys.org cover the topic of crystallization fouling, a widespread phenomenon where scale forms on surfaces. Its impact stretches across the fields of energy and water.
Despite earlier research, there remains a fundamental gap in the understanding of how microfoulants attach in ever-changing watery environments. This issue impedes the development of intentionally designed surfaces that inherently resist such phenomenons.
In a recently published study in Science Advances, Julian Schmid and a group of researchers -based both in Switzerland and the United States- focused on the interrelational dynamics of microfoulants. They used a micro-scanning fluid dynamic gauge system to manifest a rationally constructed coating that can eliminate 98% of deposits, especially under shear flow conditions.
Water and energy are interlinked, where water is crucial in generating energy for transportation, water treatment, and desalination. However, these finite resources face growing pressures, such as population growth and climate change. Researchers have looked at alternatives, including interfacial materials, surface engineering, and coatings, to tackle the issue of scale formation passively. These methods are not only sustainable but also cost-efficient.
In the past, investigations have focused on creating rigid antifouling surfaces that change the material's surface energy to eradicate fouling. Materials scientists increasingly concentrate on creating interfacial materials and coatings that amplify antifouling traits by using the inherent barriers of the materials.
In their study, Schmid and his team devised a new technique to study microfoulant adhesion physics. The team developed a micro-scanning fluid dynamic gauge, unearthing three core mechanisms of microfoulant expulsion. They designed a microtextured coating and assessed its scalability under diverse flow conditions. The results may enable better designs of antifouling surfaces and provide insights into the properties of crystallization and particulate fouling, thereby addressing the challenges of the water–energy nexus.
Furthermore, the team examined how to remove crystallites before the formation of persistent scale layers by observing the dynamics of super-wettability and transport systems found in nature, such as the interaction between crystallite-water. The studies provided insights into the development of bioinspired repellant substrates.
The fast removal process of crystallites has implications for the development of antifouling or scalephobic materials. For a broader understanding of these materials, the team replaced complex crystallite scales with spherical polystyrene microparticles and studied the varying effects on shear, modulus, wettability, and thickness.
Another method utilized by Schmid and his team involved using particulate fouling by settling microfoulants on the coating. This approach was especially helpful for microfoulants that were smaller or larger than the coating thickness. It allowed further experiments to detect interactions between the microfoulant and the coating.
In conclusion, the researchers decided to increase the polymer content of the coating by 50% based on previous research results. Nonetheless, this increase had adverse effects on the adhesion and removal properties of the coating.
The outcomes highlighted the excellent scale-phobicity of the coating. For instance, the first crystallites were removed from the microtextured polymer almost immediately after initiating flow. From the outset, the team removed a substantial number of crystallites to obtain a near clean surface to highlight the properties of scalephobicity of the designed coating under turbulent flow conditions.
In this way, Julian Schmid and team incorporated adhesion and interfacial fluidic theories to develop a method to study the underlying physics of microfoulant adhesion on and removal from engineered materials. They developed this technique based on a previously established method to analyze antifouling materials to improve the approach to study antifouling.
The method provided insights into the dynamics of the full behavior of the coating. The outcomes revealed the interactions between foulants, substrates and water to remove surface adhered crystallites under flow conditions. The team explored the versatility of antifouling materials and how the design strategies varied depending on the dominant fouling mechanism.
For instance, with particulate fouling, rigid coating surfaces performed well, whereas soft coating outperformed with crystallization fouling. Hydrogels on the other hand had a low polymer content and therefore showed excellent removal performance for both microfoulants and crystallites. For nonporous and hydrophilic hydrogels, the polymer content had to be increased, which Schmid and team mitigated by microtexturing the surface.
The materials scientists realized intrinsically scalephobic surfaces and microtextured soft hydrogels to remove predominant regions of crystallites. The outcomes provide significant details for designing antifouling and scalephobic surfaces for adhesion and interfacial transport research under heat transfer and flow conditions.
Journal information: Science Advances
© 2024 Science X Network