Unlocking Magnetic Mysteries: High-Harmonic Probes Mastery of Electron Spin
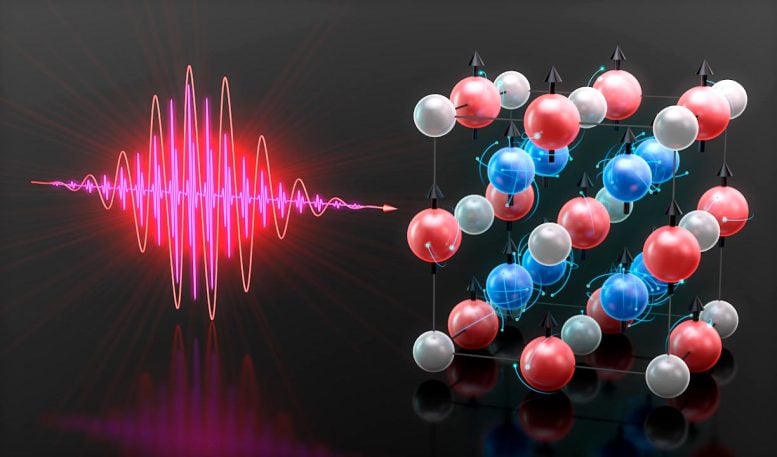
A groundbreaking study has discovered a method to gain precise control over electron spins in magnetic materials, potentially paving the way for faster, more efficient electronics. The study, headed by Margaret Murnane and Henry Kapteyn, physics professors at University of Colorado Boulder and JILA Fellows, could re-imagine the prospects for electronics and data storage.
In every piece of magnetic material, electrons move in tune with the unseen rhythm of quantum mechanics. Their spins act like minuscule atomic tops, governing the magnetic behavior of the material they are part of. The dancers in this tiny ballet are the foundation of magnetic phenomena and such spins are what the team of researchers from JILA have learned to control with striking precision.
According to a recent paper published in Science Advances, the team, along with collaborators from universities in Sweden, Greece, and Germany, investigated the spin dynamics in a Heusler compound, a type of special material that behaves as a single magnetic material, but is made up of various metals. In this study, a compound of cobalt, manganese, and gallium was studied, with the compound behaving as a conductor for electrons with upward aligned spins and as an insulator for those with downward aligned spins.
The team employed a form of light known as extreme ultraviolet high-harmonic generation (EUV HHG) to track spin reorientations within the compound. After exciting the compound with a femtosecond laser, which altered the compound’s magnetic properties, they were able to accurate measure the spin reorientations by adjusting the color of the EUV HHG light. “In the past, people haven’t done this color tuning of HHG", said JILA graduate student and co-first author Sinéad Ryan. This marked the first time a team tuned their EUV light probe across the magnetic resonances of each element inside the compound, in order to track the spin changes down to a timescale of femtoseconds.
Going one step further, the team altered the amount of power used to affect the spins. “We also changed the laser excitation fluence, or the amount of power we used to manipulate the spins", Ryan revealed, noting that this had never been done before. This allowed the researchers to manipulate the spin changes in the compound. Working together with theorist and co-first author Mohamed Elhanoty of Uppsala University, the researchers were able to verify the validity of their experimental data with theoretical models of spin changes. "We felt we'd set a new standard with the agreement between theory and experiment," Ryan asserted.
To delve deeper into the spin dynamics of their Heusler compound, the researchers used extreme ultraviolet high-harmonic probes. Ryan adjusted these probes to align with the energies of the cobalt and the manganese within the sample. This allowed the team to measure specific spin dynamics and magnetic behaviors, which could then be further manipulated.
The study revealed that by adjusting the power of the excitation laser and the photon energy of their EUV probe, the researchers could determine the dominant spin effect at different instances within the compound. They compared their measurements to a complex computational model known as the time-dependent density functional theory (TD-DFT), which predicts the changes in an electron cloud in a material when exposed to varying inputs.
Using the TD-DFT framework, Elhanoty found agreement between the model and the experimental data due to competing spin effects within the Heusler compound: spin flips up or down and spin transfers. The spin flips happen within one element in the sample as the spins shift their orientation from up to down and vice versa. In contrast, spin transfers happen within multiple elements, in this case, cobalt and manganese, as they transfer spins between each other, causing each material to become more or less magnetic as time progresses. “What he [Elhanoty] found in the theory was that the spin flips were quite dominant on early timescales, and then the spin transfers became more dominant,” explained Ryan. “Then, as time progressed, more de-magnetization effects take over, and the sample de-magnetizes.”
Understanding which effects were dominant at which energy levels and times allowed the researchers to understand better how spins could be manipulated to give materials more powerful magnetic and electronic properties.
“There’s this concept of spintronics, which takes the electronics that we currently have, and instead of using only the electron’s charge, we also use the electron’s spin,” elaborated Ryan. “So, spintronics also have a magnetic component. Using spin instead of electronic charge could create devices with less resistance and less thermal heating, making devices faster and more efficient.”
From their work with Elhanoty and their other collaborators, the JILA team gained a deeper insight into spin dynamics within Heusler compounds. Ryan said: “It was really rewarding to see such a good agreement with the theory and experiment when it came from this really close and productive collaboration as well.” The JILA researchers are hopeful to continue this collaboration in studying other compounds to understand better how light can be used to manipulate spin patterns.