Giant anomaly in Earth's mantle possibly leftover from collision that created moon
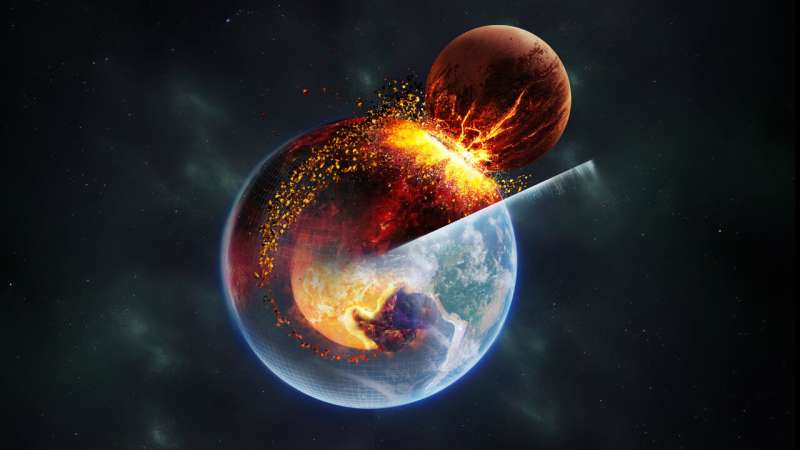
November 1, 2023
In accordance with the editorial process and policies of Science X, this article has been thoroughly reviewed. Some key aspects that were validated to maintain the credibility of the content include:
- Facts have been checked
- The article has been peer-reviewed
- Sourced from a trusted source
- Proofread thoroughly
Reviewed by the Chinese Academy of Sciences
Recently, an interdisciplinary team of international scientists discovered a significant anomaly in the Earth's core. They hypothesize it to be a remnant from the ancient collision that formed the moon around 4.5 billion years ago.
These findings present a significant step in understanding the structure and long-term development of the Earth while providing insights into the creation of the inner solar system.
Prof. Deng Hongping, from the Shanghai Astronomical Observatory of the Chinese Academy of Sciences, used computational fluid dynamics methods to lead the study. The study was featured on the cover of Nature on 2nd November.
The origin of the moon has puzzled scientists for generations. A popular theory suggests that during the Earth's final growth stage about 4.5 billion years ago, a 'giant impact' occurred. This collision was between a Mars-sized proto-planet Theia and the primitive Earth or Gaia, creating the debris that formed the moon.
Numerical simulations imply that the moon's material predominantly originated from Theia while Gaia, due to its larger mass, contained a minimal amount of Theian material. Since these two celestial bodies formed independently and were made of different substances, the Earth (dominated by Gaian material) and the moon (governed by Theian substances) should have different compositions. However, this theory was questioned when sophisticated isotope measurements disclosed that the Earth and the moon have strikingly similar compositions.
Various refined models explaining this 'giant impact' have been brought forth over time, yet all have faced challenges.
In an attempt to offer a clear explanation, Prof. Deng, in 2017, started studying the moon's formation. He developed a cutting-edge computational fluid dynamics method called Meshless Finite Mass (MFM). This method is particularly efficient in portraying turbulence and material mixing accurately.
After conducting several simulations, Prof. Deng found that the early Earth presented mantle stratification, the upper and lower mantles had different compositions post the impact. A magma ocean, a thorough mix of Gaian and Theian material, was formed in the upper mantle. Meanwhile, the lower mantle remained mainly solid and retained the material composition of Gaia.
According to Prof. Deng, previous studies overly focused on the structure of the debris disk (which later formed the moon) and neglected the impact of the 'giant collision' on early Earth.
After discussing with geophysicists from the Swiss Federal Institute of Technology in Zurich, Prof. Deng and his team hypothesized that this mantle stratification might still exist. It could correspond to the global seismic reflectors in the mid-mantle (approximately 1,000 km beneath the Earth's surface). Prof. Deng's previous research suggests that the elemental composition (including higher silicon content) of the lower mantle differs from the upper shell, as the former still contains pre-impact Gaian material.
Prof. Deng reflected, 'Our findings challenge the traditional belief that the giant impact led to the homogenization of the early Earth. Instead, it appears that this moon-forming giant impact is the origin of the early mantle's heterogeneity and marks the onset of Earth's geological evolution which has spanned over 4.5 billion years.'
Another example of Earth's mantle heterogeneity is two anomalous regions—called Large Low Velocity Provinces (LLVPs)—that stretch for thousands of kilometers at the base of the mantle. One is located beneath the African tectonic plate and the other under the Pacific tectonic plate. When seismic waves pass through these areas, wave velocity is significantly reduced.
LLVPs have significant implications for the evolution of the mantle, the separation and aggregation of supercontinents, and the Earth's tectonic plate structures. However, their origins have remained a mystery.
Dr. Yuan Qian from the California Institute of Technology, along with collaborators, proposed that LLVPs could have evolved from a small amount of Theian material that entered Gaia's lower mantle. They subsequently invited Prof. Deng to explore the distribution and state of Theian material in the deep Earth after the giant impact.
Through in-depth analysis of previous giant-impact simulations and by conducting higher-precision new simulations, the research team found that a significant amount of Theian mantle material, approximately 2% of Earth's mass, entered the lower mantle of Gaia.
Prof. Deng then invited computational astrophysicist Dr. Jacob Kegerreis to confirm this conclusion using traditional Smoothed Particle Hydrodynamics (SPH) methods.
The research team also calculated that this Theian mantle material, similar to lunar rocks, is enriched with iron, making it denser than the surrounding Gaian material. As a result, it rapidly sank to the bottom of the mantle and, over the course of long-term mantle convection, formed two prominent LLVP regions. These LLVPs have remained stable throughout 4.5 billion years of geological evolution.
Heterogeneity in the deep mantle, whether in the mid-mantle reflectors or the LLVPs at the base, suggests that the Earth's interior is far from a uniform and 'boring' system. In fact, small amounts of deep-seated heterogeneity can be brought to the surface by mantle plumes—cylindrical upwelling thermal currents caused by mantle convection—such as those that likely formed Hawaii and Iceland.
For example, geochemists studying isotope ratios of rare gases in samples of Icelandic basalt have discovered that these samples contain components different from typical surface materials. These components are remnants of heterogeneity in the deep mantle dating back more than 4.5 billion years and serve as keys to understanding Earth's initial state and even the formation of nearby planets.
According to Dr. Yuan, 'Through precise analysis of a wider range of rock samples, combined with more refined giant impact models and Earth evolution models, we can infer the material composition and orbital dynamics of the primordial Earth, Gaia, and Theia. This allows us to constrain the entire history of the formation of the inner solar system.'
Prof. Deng sees an even broader role for the current study. 'This research even provides inspiration for understanding the formation and habitability of exoplanets beyond our solar system.'
Journal information: Nature
Provided by Chinese Academy of Sciences