Revealing Ancient Mysteries: Neutron Imaging Unveils Concealed Secrets of Fossils and Artifacts
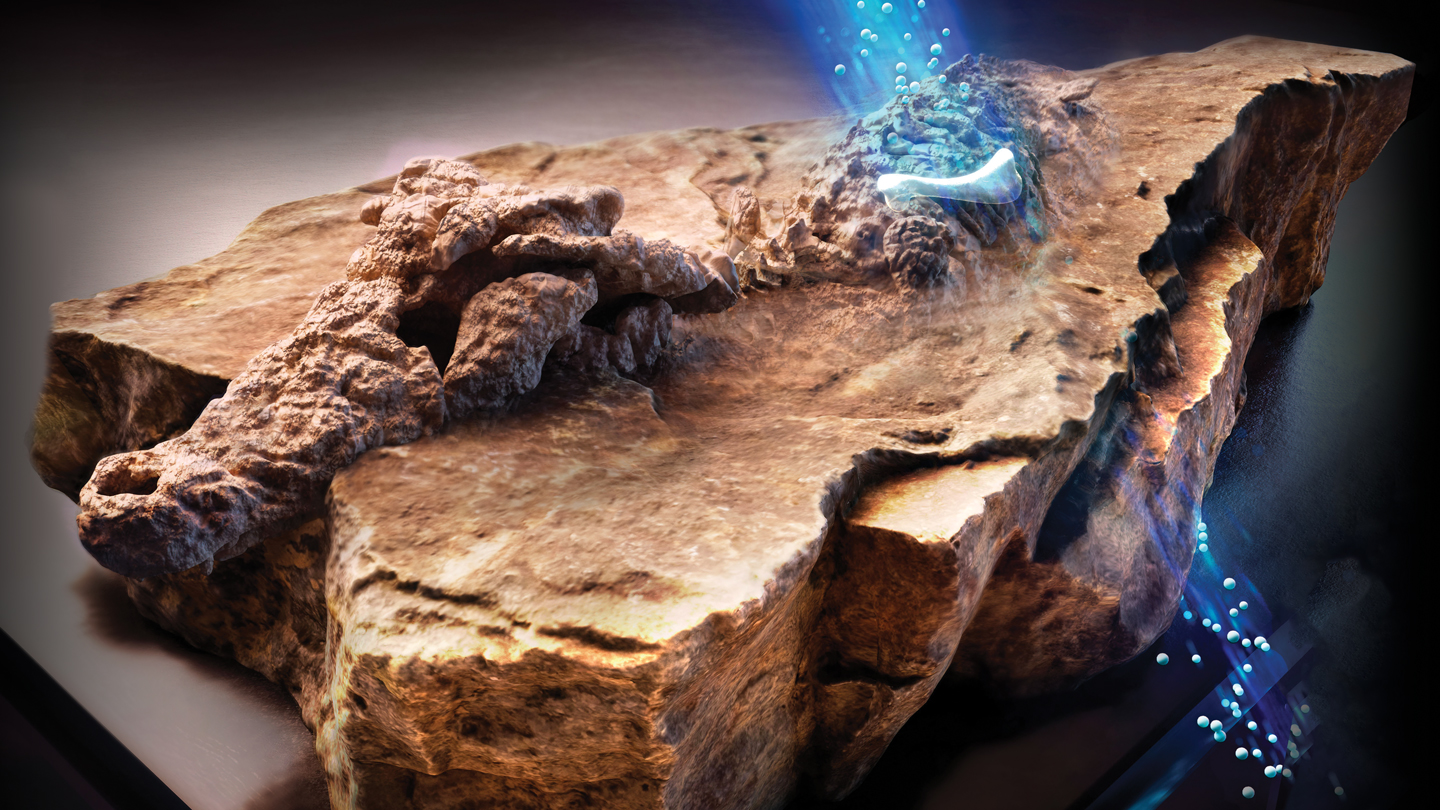
The shattered crocodile, scientifically known as Confractosuchus, was discovered in Australia. A bulldozer clearing a boulder incidentally broke a stone, which upon examination, held fossils. However, there was no hint at the time that this find would provide a rare glimpse into the Cretaceous Period.
With the help of his colleagues, Paleontologist Matt White from the University of New England in Armidale, Australia arranged for the stone to be scanned using X-ray computed tomography. Similar to a clinical CT scan, this method generates multiple images that can be compiled to form a 3-D blueprint of the object's interior. They planned to use these scans to isolate the separate bones without extracting them, then rearrange the 3-D representations to reconstruct the shattered crocodile digitally.
However, the team encountered difficulty with one section of the fossil. The presence of iron-rich stone around the bones interfered with the quality of the X-ray images. This led the researchers to explore an alternate approach.
The bone fragment causing issues was sent to chemist Joseph Bevitt of the Australian Centre for Neutron Scattering in Sydney, who is experienced in using subatomic neutron particles for imaging ancient objects. Besides the anticipated croc bones, Bevitt found one that seemed to resemble a dinosaur leg bone. The location of this bone corresponded to where the crocodile's stomach cavity would have been.
Bevitt shares his surprise and shock on uncovering the dinosaur femur in the neutron imaging result. Further X-ray and neutron scanning, along with several years of analysis ultimately confirmed the presence of an unknown dinosaur species' remains, bearing tooth marks and shredded into pieces, within the crocodile's stomach. This revelation is what led to the "sauroktonos" - translating to lizard killer, being added to the shattered crocodile's name. The discovery of the new species of crocodile and its unusual prey was made public in Gondwana Research in 2020.
The unique case of Confractosuchus sauroktonos—the shattered croc, the lizard predator—and the remnants of its last dinosaur meal, encased in stone for roughly 100 million years, is an extraordinary find. These profound insights would not have been possible without neutron tomography. The usage of neutron particles for imaging purposes has been happening for decades in industries and militaries, and it is only recently that these particles have proven useful for analyzing fossils and antiquities with unprecedented detail.
Earlier, studying fossils and artifacts often led to their damage or absolute destruction. Moth-eaten remains were dissected, sealed containers were forcefully opened, fossils were prised loose from stones. In some instances, fossil-holding samples had to be progressively ground to form photographs of the sections, revealing the fossilized structures within. Non-destructive methods have come into play with the advent of X-rays.
X-rays, being a form of high-energy electromagnetic radiation, interact with electrically charged particles' electric and magnetic fields. When an X-ray is directed at a broken bone, light is either absorbed or scattered by the electrons surrounding the leg's atoms. Materials with higher density pack in more electrons, causing X-rays to pass through less effectively, thus, these parts appear more prominently in X-ray images.
Ever since the discovery of this radiation in 1895, X-rays have helped us peer into artifact interiors. However, with the advent of computing power in the 1970s, X-ray CT became the standard method for examining objects in the fields of paleontology and archaeology. Recent uses of X-ray CT scanning include a study of brain cavities in a 20-million-year-old monkey skull and scanning mummified animals from ancient Egypt. Most major museums and research institutions have their own similar systems to the ones doctors use for X-ray CT scanning.
X-ray imaging, despite its revelations about the past, still has its shortcomings. The inability of X-rays to penetrate dense materials such as lead or thick layers of other metals makes it impossible to peers inside such objects. Similarly, objects made of low-density material, like soft tissue, remain undetectable to X-rays.
This is where neutrons come into play.
Neutrons, living up to their name, are neutral. These subatomic particles lack an electric charge, as a result, they do not interact with the electrons orbiting atoms. Instead, neutrons bypass electrons and target nuclei packed with protons and neutrons at the center of atoms. As they approach, neutrons can either bounce off an atom's nucleus or be absorbed by it. These interactions, governed by the speed of neutrons and intricate quantum mechanical interactions, are more complex than that of X-rays.
Neutrons fit for tomography are produced using relatively huge particle accelerators or as by-products from nuclear reactors. These neutrons are relatively slow and their energies are a hundred-millionth of those of X-rays in CT scanners. These slow neutrons strongly interact with some low-density materials which X-rays effortlessly pass through, including lithium, boron, and hydrogen.
Bevitt likens water's interaction with neutrons to lead's interaction with X-rays due to the hydrogen atoms. An excess of hydrogen-rich material can obstruct details from neutron beams. However, akin to how a metal hip joint stands out in a medical X-ray, hydrogen can also highlight some features in neutron images. Metals like lead, iron, and copper are essentially invisible to low-energy neutrons.
Physicist Jacob LaManna from the National Institute of Standards and Technology in Gaithersburg, Md., exemplifies the comparative efficiencies of neutron and X-ray imaging with a CT “still life” of Asiatic lilies within a hollow cask encased with thick lead walls. “The neutrons can pass through the lead, and then one can see practically all the water [inside] the vascular structure of the flowers,” explains LaManna. An X-ray scan, on the other hand, would only reveal the opaque outer shell of the cask.
Neutron imaging, due to its capability of penetrating dense materials that obstruct X-rays, has become integral to the industrial examination of automobiles and aircraft. The particles can display the circulation of hydrogen-rich oil inside engine blocks or expose flaws in metal castings. Since the 1970s, U.S. national laboratories have used neutron imaging to develop and preserve the nation's nuclear weapons stockpiles; neutron serve as effective quality assurance tools for visualizing the interiors of dense bomb parts and for scrutinizing hydrogen-rich fusion explosives within warhead components.
At NIST, LaManna supervises the Neutron and X-ray Tomography, or NeXT, facility, capable of functioning X-ray and neutron imaging simultaneously. This dual view approach offers distinct but complementary data about items that contain a mix of materials - such as hydrogen fuel cells, building materials, and soil samples - that would be challenging to research with only one type of imaging method.
Neutron imaging, over the past few decades, as awareness about its potentialities has proliferated, has been increasingly adopted by paleontologists, archeologists, and anthropologists as an analytical tool. Despite its long-standing history, as Bevitt puts it, "we are really the new kids on the block."
Neutron computed tomography, in addition to uncovering numerous dinosaur bones inside a shattered crocodile, along with the femur that originally caught Bevitt’s attention, has facilitated researchers to study fabric enveloping cat mummies without unpacking them, discover traces of recent glues securing fraudulently put together artifacts, and excavate the oldest-ever vertebrate heart discovered in a 380-million-year-old fish.
Paleontologist James Clark has two fossilized crocodile skulls on the table in his basement lab at George Washington University in Washington, D.C. The 165-million-year-old fossils are overshadowed by a nearby contemporary alligator skull. While the alligator skull roughly has the same length as a forearm, the fossilized croc skulls are slightly bigger than a thumb tip.
The delicate skulls, which Clark collected from Mexico forty years ago, are incased in surdified pieces of sediment with only a few bones and teeth visible. On first sight, the specimens look like lumps of chewed gum, but are composed of gritty, iron-rich mudstone. “If you attempt to X-ray that, one would essentially wind up with … bright sparks from all the iron,” asserts Clark. The outcome is a blurry and streaked image that obscures the skeletal structures.
Clark could have assigned preparers to eliminate the sediment enveloping the fragile bones. However, he points out that the process is time-consuming and costly, and can potentially damage the specimen.
In 2019, he was finally able to examine the concealed bones. Upon meeting Bevitt at a seminar, Clark began to understand the potential of neutron scanning. This encounter also introduced him to LaManna and the NIST facility positioned 25 kilometers away in Maryland.
LaManna notes that iron is almost completely transparent to neutrons, which makes it much simpler to focus solely on the fossil part of the object. The intricate details of the small bones were revealed by images from the NIST neutron CT scans. This led to the possibility of digitally reassembling the bone fragments to recreate the original creature.
Issues arise when the material surrounding a fossil or object presents a challenge for X-rays. Items like tissues, fibers, wood, and other low-density substances can be hard to detect with X-rays because metals within the object can obstruct the view. This problem is encountered by researchers studying ancient artifacts like 3,000-year-old dagger-axes, which are displayed in the Freer Gallery of Art in the Smithsonian, located in Washington, D.C.
These ceremonial weapons from China’s Shang dynasty are showcased in a vertical glass case in which you can get up close with the jade blades and bronze handles adorned with turquoise. For a more detailed view, one must get closer to appreciate the intricate patterns of gemstones nestled in the metal.
Ariel O’Connor, a conservator at the Smithsonian, is intrigued by how the dagger-axes were constructed. Traditional X-rays cannot penetrate the combination of stone, metal, fibers, and other materials present in these artifacts. While neutron imaging could be the solution, it also presents a risk - it affects the radioactivity of objects. The radioactivity of a sample isn't always predictable and often exceeds safety levels for human handling or viewing post exposure.
LaManna says calculations can be made to predict the concerning elements and estimate the duration of radioactivity. However, unknown materials in the jade make accurate predictions challenging.
To test this, O’Connor and her colleagues created a crude imitation of an ancient dagger-ax. They used Wyoming jade, brass from a repurposed door kickplate, and a silk thread similar to those connecting Shang dynasty dagger-axes. Then, LaManna conducted X-ray and neutron scans at NIST.
The X-ray could not penetrate the brass, hiding the dagger’s structure. However, the neutron beam unveiled key details, even revealing the jade inside the brass handle and the individual silk threads.
To examine the effectiveness of neutron imaging on a dagger-axe, the team built a replica using jade, brass, and silk thread. While the X-ray failed to penetrate the brass, neutron scanning unveiled the inner details.
Nine days after the procedure, the replica showed no significant residual radioactivity. Bevitt states that generally, residual radiation fades quickly, with most samples safe for handling within a few weeks or less. However, when radium was involved in one experiment, the sample remained radioactive for three months.
Nevertheless, due to the associated risks and uncertainties, O’Connor is hesitant to scan real artifacts. As a conservator, she is responsible for the preservation and safety of these treasures and prioritizes preventing any alterations that may render them inaccessible due to radioactivity.
Although more researchers are now using neutron tomography to examine fossils and antiquities, X-ray CT remains the preferred method. In the 1990s, a handful of scholarly articles were published each year on the use of neutrons in historical research. Nowadays, it's grown to hundreds of publications annually. However, this number is dwarfed by the thousands of articles published every year on the use of X-ray CT to study fossils and artifacts.
Most of the time, X-rays suffice, and the advantages are clear. They offer high resolution to uncover small details with no lingering radioactivity. X-ray CT machines are also widely available because they’ve been used in medical settings for over 50 years, and they’re small enough to fit in most labs and museum research spaces.
At the moment, there are only a few dozen neutron tomography facilities on the planet. The particle accelerators and nuclear reactors that produce suitable neutrons are large, expensive and heavily regulated. Only a handful of the facilities worldwide are available to analyze fossils and antiquities, according to Burkhard Schillinger, a physicist at the Technical University Munich who runs the neutron imaging beamline there. He ticks off a few facilities in the United States, a half dozen in Europe and one in Australia.
Still, LaManna says the lack of access doesn’t seem to be the bottleneck in widespread adoption of the technique. Along with the concerns over lingering radioactivity, the novelty of the technology and general lack of awareness may stand in the way.
“I try to recruit as broad a range of users as I can” to submit fossils and antiquities for imaging at NIST, LaManna says. “It’s not like they’re getting pushed out of the way” to make space for more conventional neutron studies. “It’s just more of getting the correct people interested to then write proposals, come to us [and] work with us to get beam time.”
In the last decade, Australia-based Bevitt has spread the word on neutron tomography through lectures and outreach around the world. Most of the experts contacted for this story trace their initial interest in neutron imaging to Bevitt’s influence. Many researchers in his home country have already embraced the technology.
“Basically, in Australia, when a new dinosaur is discovered,” Bevitt says, “the first thing that happens is it comes to our lab.”
This article was supported by readers like you. Invest in quality science journalism by donating today.