Reimagining Carnot: Breakthrough in Balancing Power and Efficiency by Scientists
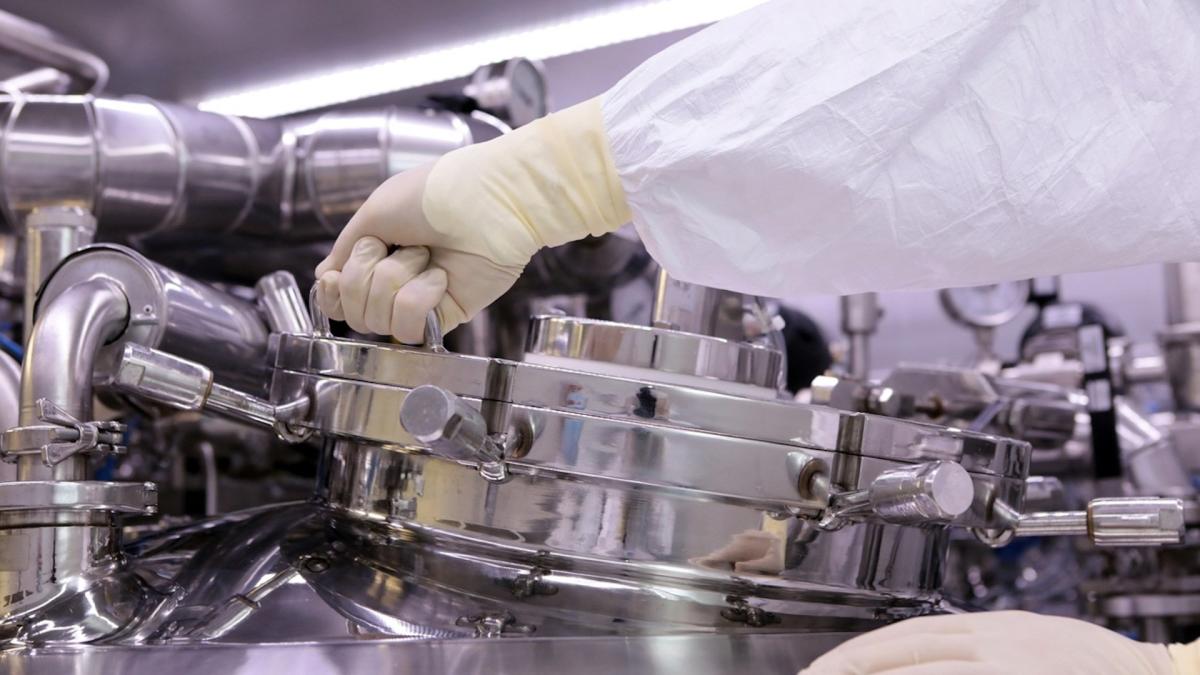
January 31, 2025
feature
This article has been reviewed according to Science X's editorial process and policies. Editors have highlighted the following attributes while ensuring the content's credibility:
- fact-checked
- peer-reviewed publication
- trusted source
- proofread
by Tejasri Gururaj, Phys.org
Challenging centuries-old assumptions about thermodynamics, a new study in published in Physical Review Letters has shown that it is theoretically possible to design a heat engine that achieves maximum power output while approaching Carnot efficiency.
The Carnot heat engine is a thermodynamic device that converts heat into mechanical work by operating between two temperature reservoirs, a hot and cold one.
The engine works by taking heat from the hot reservoir, converting some of it into useful work, and rejecting the remaining heat to the cold reservoir. The thermodynamic cycle followed by the engine is known as the Carnot cycle.
In an ideal case, this process would be perfectly reversible and the Carnot engine would have maximum efficiency. In reality, real heat engines are not reversible and lose energy in the form of heat.
Thus, the efficiency of a real heat engine is always less than that of a Carnot heat engine operating between the same reservoirs. This is the second law of thermodynamics.
The challenge with building a heat engine that has an efficiency close to the Carnot heat engine is it takes infinite time to do the work with minimal power output.
The researchers addressed this problem with a biochemical heat engine. Phys.org spoke to the co-authors of the study, Assistant Prof. Yu-Han Ma from Beijing Normal University and Dr. B. Shiling Liang from the Center for Systems Biology Dresden.
Prof. Ma said, 'This collaborative work originated from a discussion between Shiling and me at the end of 2022. At that time, in his early explorations, Shiling discovered that degeneracy could increase the efficiency at maximum power (EMP) of heat engines.'
Dr. Liang added, 'Drawing from a polymer folding model in my previous work, I developed a minimal heat engine that, surprisingly, showed the potential to exceed some accepted limits on heat engine efficiency at maximum power. This unexpected finding led me to reach out to Yu-Han, initiating our collaboration on this project.'
Heat engines, which convert thermal energy into useful work, have been fundamental to human civilization since the Industrial Revolution.
However, they've always faced a seemingly insurmountable trade-off when it comes to reaching the Carnot efficiency. They could either operate at maximum efficiency by moving very slowly (essentially producing no power) or generate useful power by sacrificing efficiency.
The most notable is the '1/2-universality principle.' According to this, heat engines operating in the linear response regime (small temperature differences) can only achieve half the Carnot efficiency at maximum power.
'This trade-off relation has been proven to be universal in various situations, especially in low-dissipation heat engines, where the efficiency at maximum power has a clear upper limit, and there is a significant gap between it and the Carnot efficiency,' explained Prof. Ma.
The solution came in the form of a system with degenerate energy levels, meaning that each energy level has different microscopic states or configurations, all corresponding to the same energy level.
The model consists of two states, a low and high-energy state, with the higher energy state capable of accommodating many more molecular configurations, i.e., a higher degeneracy.
There are two reaction pathways for transitions to occur between the energy levels. An ATP-hydrolysis-drive reaction occurs at low temperatures and spontaneous transition happens at high temperatures.
At high temperatures, the system naturally tends toward the high-energy state because it can access the many possible configurations available in this state. This makes the spontaneous transition more likely to occur, and this pathway doesn't require ATP.
On the other hand, at lower temperatures, the ATP-hydrolysis-driven reaction is more likely and can drive the system from the low-energy state to the high one.
As the system size increases, meaning the high-energy state can accommodate more and more configurations compared to the low-energy state, the transitions become more sharp or switch-like. These types of transitions are known as first-order phase transitions and happen with minimal energy loss.
'By constructing a minimal model incorporating this feature, we could demonstrate how it breaks conventional thermodynamic bounds and reveal the physical mechanism behind collective advantage,' explained Dr. Liang.
Discover the latest in science, tech, and space with over 100,000 subscribers who rely on Phys.org for daily insights. Sign up for our free newsletter and get updates on breakthroughs, innovations, and research that matter—daily or weekly.
The researchers demonstrated that their biochemical engine can achieve Carnot efficiency while maintaining maximum power output as the system size approaches infinity. The power scales linearly with system size while efficiency approaches the Carnot limit.
Dr. Liang spoke of the design principle of their model, 'Engineering systems with high-degeneracy states can significantly enhance heat engine performance.'
'This is similar to how the Carnot engine, while idealized, has guided the development of practical heat engines for centuries. Even if perfect degeneracy isn't achievable, we now know that increasing degeneracy can be a valuable strategy for developing more efficient heat engines.'
The study also shows the violation of a well-established universality.
Prof. Ma explained, 'Our research shows this universality is violated in the limit of large degeneracy.'
'The order of taking the limits of the Carnot efficiency and the degeneracy affects the proportionality coefficient of the EMP with respect to the Carnot efficiency. This implies that when the system has a diverging intrinsic quantity, some conventional thermodynamic constraints may need to be re-examined.'
Further, the system operates as a biochemical engine that can synthesize ATP, making it relevant for biological systems. The next step would be to find practical heat engines with these properties, which is a challenge. Dr. Liang pointed out that biopolymers are promising candidates, as they naturally possess highly degenerate unfolded states.
More information: Shiling Liang et al, Minimal Model for Carnot Efficiency at Maximum Power, Physical Review Letters (2025). DOI: 10.1103/PhysRevLett.134.027101. On arXiv: DOI: 10.48550/arxiv.2312.02323
Journal information: arXiv, Physical Review Letters
© 2025 Science X Network