Unveiling MXenes: Pioneering Atomic-Level Insights Reveal Limitless Potential
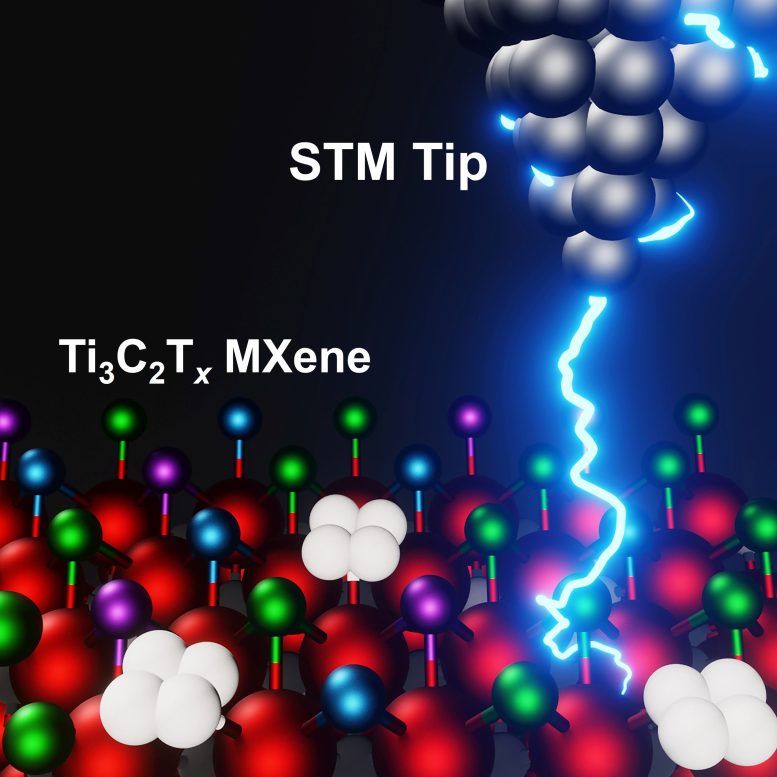
Using scanning tunneling microscopy, researchers at Drexel University and UCLA are providing the first atom-scale look at the surface of 2D MXene materials. The findings will help to tailor the unique materials for specific applications. Credit: Drexel University
Advanced imaging techniques have revealed the complex surface chemistry of MXenes, a promising material for energy and telecommunications applications, potentially leading to customized functionalities for specific uses.
In the decade since their discovery at Drexel University, the MXene family of two-dimensional materials has demonstrated significant potential for applications ranging from water desalination and energy storage to electromagnetic shielding and telecommunications, among others. While the origins of their versatility have been widely speculated upon by researchers, a recent study led by Drexel University and the University of California, Los Angeles, has offered the first clear insight into the surface chemical structure that underpins MXenes’ capabilities.
Using advanced imaging techniques, known as scanning tunneling microscopy (STM) and scanning tunneling spectroscopy (STS), the team, which also includes researchers from California State University Northridge, and Lawrence Berkeley National Laboratory, mapped the electrochemical surface topography of the titanium carbide MXene — the most-studied and widely used member of the family. Their findings, published in the 5th-anniversary issue of the Cell Press journal Matter, will help to explain the range of properties exhibited by members of the MXene family and allow researchers to tailor new materials for specific applications.
“Much of MXenes’ potential results from their rich surface chemistry,” said Yury Gogotsi, PhD, Distinguished University and Bach professor in Drexel’s College of Engineering, a lead author of the research, whose research group participated in the materials’ discovery in 2011. “Getting the first atomic-scale look at their surface, using scanning tunneling microscopy, is an exciting development that will open new possibilities for controlling the material surface and enabling applications of MXenes in advanced technologies.”
Although MXenes are two-dimensional materials, the interaction that is the basis of their chemical, electrochemical, and catalytic properties — whether it’s ultrafast storage of electrical energy, splitting water to produce hydrogen, or skimming urea out of blood — is initiated by the atoms that form their surface layer.
Previous research has provided a lower-resolution look at the chemical structure of MXene surfaces, using technology such as scanning electron microscopy (SEM), secondary ion mass spectroscopy (SIMS), and tip-enhanced Raman spectroscopy (TERS). These tools offer indirect readings of the material’s composition, but provide little information about the intricacies of its surface organization.
Scanning tunneling microscopy and scanning tunneling spectroscopy, by contrast, provide more direct information about the shape and composition of a material’s surface structure, as well as its surface chemistry and properties.
These tools use an extremely sharp probe, sensitive enough to distinguish one atom from another as it scans across a flat surface. The tip of the probe carries an electric charge that enables it to interact with each atom as it passes by, this interaction — called quantum tunneling — provides information about the atoms on the surface of the material. Spectroscopic scans provide information about surface composition at the atomic and molecular levels. The scans are converted into images, forming topographical maps of the material’s surface.
“With STM/STS, we can see atomic arrangements on MXenes’ surface and even study their conductance with atomic resolution,” Gogotsi said. “This is the key to understanding why MXenes have extreme properties and outperform other materials in many applications. It should also help us to explore quantum properties of MXenes and identify new opportunities for this quickly expanding family of materials.”
Locating groups of atoms — called functional groups — identifying them and measuring their properties on the surface, given their specific location and attachment, are all important developments for understanding how MXenes interact with other chemicals and materials, according to the researchers.
“The MXene surfaces are chemically heterogeneous. That is both what makes them interesting and what makes them difficult to study,” said Paul Weiss, PhD, a distinguished professor and UC Presidential chair at UCLA who led the research with Gogotsi. “We believe that it is also key to their amazing properties. However, we do not yet know which chemical functionalities are important for which applications.”
The group’s STM/STS imaging showed 10-nanometer features on the MXene’s surface, likely to be titanium oxide clusters, and smaller protrusions, arrayed in a distorted hexagonal symmetry, that they deemed to be functional groups, which they went on to identify chemically.
The results of this research were consistent with previous theories, lower-resolution microscopy, and spectral data about the surface of titanium carbide MXenes, including the prediction that their surface is metallic. However, getting a closer look at the surface defects and the nature of its heterogeneity is an important step in understanding how they affect the material’s behavior, according to the team.
“In this work, we started pulling at the threads. We were able to image and to start to assign some of the chemical functionality,” Weiss said. “One of the most interesting unknown aspects of MXenes is what roles their defects and heterogeneity play in their function and environmental stability. We now have our foot in the door to explore these roles.”
Drawing on the collective expertise of Drexel’s materials scientists, the STM groups at UCLA and Lawrence Berkley National Laboratory, and theoretical scientists at Cal State Northridge, the group will continue its rigorous analysis of the materials as it lays out a process for modulating their chemical composition to tune their functionality for different uses.