Potential 'Neutrino Fog' May Pose Challenges for Dark Matter Detection
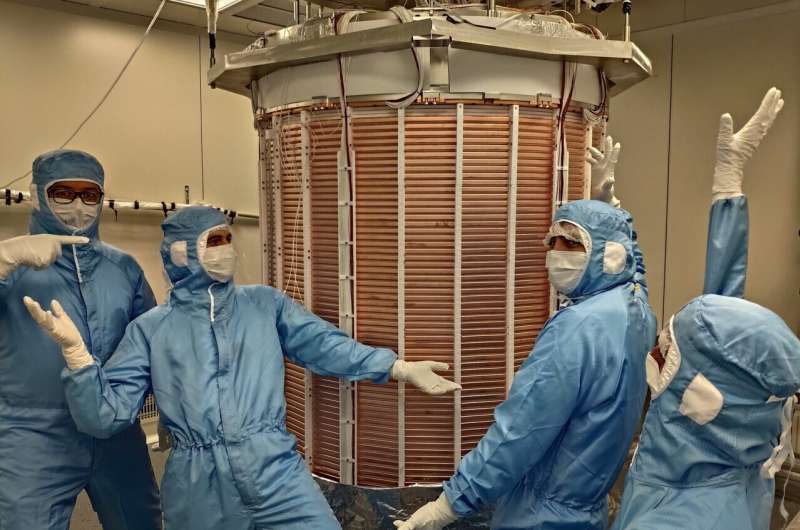
November 12, 2024 feature
This article has been reviewed according to Science X's editorial process and policies. Editors have highlighted the following attributes while ensuring the content's credibility:
- fact-checked
- peer-reviewed publication
- trusted source
- proofread
by David Appell , Phys.org
As if searching for dark matter isn't difficult enough already, physicists may have detected another hurdle known as a 'neutrino fog' from solar neutrinos streaming through Earth.
Several experiments are currently being conducted to detect dark matter, the hypothetical something that constitutes 27% of the mass-energy of the universe and would explain the rotation curves of galaxies and more. Dark matter is expected to be cold particles that are nearly non-interacting with regular matter—thus they are dubbed weakly interacting massive particles (WIMPs).
One popular hoped-for detection method is deep underground storage containers of xenon in its liquid form. The hope is that dark matter impinging on the Earth will travel through the rock above it to the detector, screening out nearly all cosmic rays that also hit the Earth.
But neutrinos are also very weakly interacting particles that can reach deep underground, and the sun provides a large flux of them—for lower energies up to 18 MeV/c2, up to 700 trillion per second on each square-meter.
Almost the entirety passes directly through the Earth, but a minuscule few will react with an atom or nucleus. As detectors get more and more sensitive, this 'neutrino fog' can interfere with their ability to detect dark matter. And it now looks likely this has been seen in two underground experiments looking for dark matter: the PandaX-4T experiment located at the China Jinping Underground Laboratory located in Sichuan and the XENONnT experiment at the Gran Sasso National Laboratory in Italy.
Both groups published papers on the same day in Physical Review Letters with evidence of neutrino interactions in their detector from nuclear recoils as a neutrino scatters from a xenon nucleus. While their results are just below the three-sigma test of significance which physicists customarily use for evidence, they do not yet approach the five-sigma standard for a discovery. Still, the results hint that the neutrino fog could well complicate their future efforts.
Solar neutrinos are produced in the sun's chain of nuclear reactions, when 8B (boron) decays into 8Be* (beryllium in an excited, unstable state), a positron and an electron neutrino. (Boron has an atomic number of 5, with five protons in its nucleus and six neutrons; 8B is a radioisotope with two more neutrons in the nucleus, and a half-life of only 772 milliseconds.)
These neutrinos, which make up only 0.02% of all solar neutrinos, easily escape the solar plasma and some stream to Earth, rudely interrupting the patient dark matter detectors. The few that scatter do so by a process called coherent elastic neutrino nucleus scattering, or CEνNS. (The Greek letter nu (ν) is the common symbol for a neutrino.)
The neutrino interacts with the nucleus as a whole and is more likely than interactions with individual nucleons or electrons. The CEνNS scattering process was discovered at an accelerator in 2017, but for the low neutrinos energies it is very difficult to observe because the recoiling nucleus has a small energy, as low as 1 keV/c2.
The detectors consist of 1–2 cubic meters of liquid Xenon with a mass of 4–6 tons, at a temperature of about -110°C (163 K), installed 1,400 meters below the surface in Italy, 2400 meters in China. The initial primary objective for both is to detect dark matter.
Each gathered data from two different experimental runs over the course of two years, searching for candidate CEνNS interactions based on theoretical predictions that include the detector performance and the known flux of 8B neutrinos.
XENONnT's detector observed 11 CEνNS events via machine-learning data analysis (and 26 events attributed to background sources), while PandaX-4T reported 75. Although XENOXnT's detector has 60% more volume and had an effective mass exposure time 60% higher, PandaX-4T is a kilometer deeper underground.
But the primary reason for PandaX-4T's larger count is a lower threshold energy for what constitutes an interaction. The downside of that lower threshold is larger background noise for the data analysis to grind through and eliminate.
Both measured a neutrino flux from the sun that, in overlap, is consistent with what the standard solar models of the sun predict—about 50 billion neutrinos/m2/s, and a scattering cross section of the CEνNS process for Xenon consistent with the Standard Model of particle physics.
In the end, each experiment obtained similar statistical confidence that they had detected 8B CEνNS interactions—2.64 sigma for PandaX-4T and 2.73 sigma for XENONnT. (A 3-sigma confidence level means the result has a 0.3% chance of being a background fluctuation; 5-sigma has only a 0.00006% chance.) As mentioned above, these aren't quite high enough for the results to be considered evidence.
'With ongoing data taking of PandaX-4T and the future upgrades, we expect to significantly improve the 8B neutrino measurement, opening exciting new science opportunities from deep underground,' the PandaX Collaboration wrote. 'The development of liquid xenon detectors may also open new avenues for low-energy neutrino detection using the CEνNS channel.'
The XENON Collaboration wrote, 'As XENONnT continues to take data, more precise measurements are expected in the future,' hopefully exceeding the 3-sigma threshold for evidence.
More information: Zihao Bo et al. (PandaX Collaboration), First Indication of Solar 8B Neutrinos through Coherent Elastic Neutrino-Nucleus Scattering in PandaX-4T, Physical Review Letters, 2024. DOI: 10.1103/PhysRevLett.133.191001
E. Aprile et al. (XENON Collaboration), First Indication of Solar 8B Neutrinos via Coherent Elastic Neutrino-Nucleus Scattering with XENONnT, Physical Review Letters (2024). DOI: 10.1103/PhysRevLett.133.191002. journals.aps.org/prl/abstract/ … ysRevLett.133.191002
Journal information: Physical Review Letters
© 2024 Science X Network