"Breakthrough in Viscous Electron Flow Enables Terahertz Photoconductivity in Graphene"
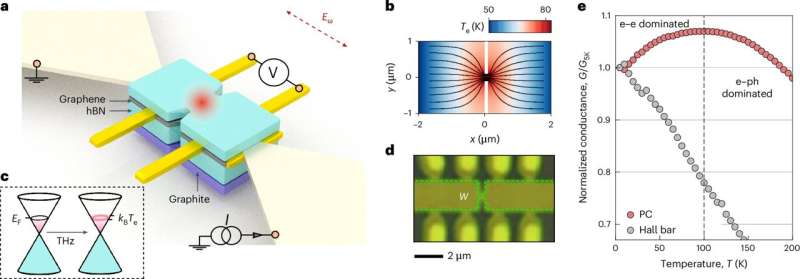
November 9, 2024 feature
This article has been reviewed according to Science X's editorial process and policies. Editors have highlighted the following attributes while ensuring the content's credibility:
fact-checked
peer-reviewed publication
trusted source
proofread
by Ingrid Fadelli , Tech Xplore
When light hits the surface of some materials, namely those exhibiting a property known as photoresistance, it can induce changes in their electrical conductivity. Graphene is among these materials, as incident light can excite electrons within it, affecting its photoconductivity.
Researchers at the National University of Singapore report a deviation from standard photoresistive behaviors in doped metallic graphene. Their paper, published in Nature Nanotechnology, shows that when exposed to continuous-wave terahertz (THz) radiation, Dirac electrons in this material can be thermally decoupled from the lattice, prompting their hydrodynamic transport.
'Our research has emerged from the growing recognition that traditional models of electron behavior don't fully capture the properties of certain advanced materials, particularly in the quantum world,' Denis Bandurin, Assistant Professor at NUS, lead of the experimental condensed matter physics lab and senior author of the paper, told Tech Xplore.
'For a long time, we've treated electrons as independent particles, resembling atoms in a gas, making the model simpler. However, many phenomena observed in quantum materials could not be explained. Recent studies, however, suggested that under certain conditions, electrons in these materials behave collectively, like a fluid, meaning they interact and 'flow' together.'
The key objective of the study by Bandurin and his colleagues was to further explore the fluid-like behavior of graphene electrons reported in recent studies. Specifically, the team tried to determine if the viscous electron flow observed in graphene could help to solve a long-standing challenge in the field of optoelectronics, namely that of detecting THz radiation.
'THz waves, which lie between microwaves and infrared on the spectrum, are difficult to detect but have huge potential applications,' said Bandurin. 'We wanted to see if the fluid-like behavior of electrons could enhance graphene's response to THz radiation, potentially creating a practical, high-speed detector for this challenging range of electromagnetic spectrum.'
To explore the effects of THz waves on the electrical conductivity of graphene, the team first prepared single-layer graphene samples 'doped' with additional electrons, which made them behave more like metals. To realize efficient sensing in these samples, the team had to process them further, as the electrical conductivity of graphene is not sensitive to heating via THz radiation.
'To address this issue, we designed our samples to have a narrow constriction which enables viscous effects to change the conductivity of the samples which were exposed to THz radiation,' explained Mikhail Kravtsov, the first author of the paper. 'Using high-precision measurement tools, we could monitor changes in electron movement and electrical resistance within the graphene as it interacted with the THz waves.'
Interestingly, the researchers observed that when the doped metallic graphene samples were under the influence of THz light, the viscosity of their fluid-like electrons dropped. This allowed the electrons to flow through the material more easily (i.e., with less resistance).
Bandurin and his colleagues captured this observed effect in a newly developed viscous electron bolometers. These devices can promise a detection of shifts in electrical conductivity at extremely high speeds.
'The most exciting achievement of our study was developing the first practical application of viscous electron flow, a concept previously seen as purely theoretical,' said Bandurin.
'By using the THz waves to alter the electron viscosity in graphene, we successfully created a device that detects THz radiation with high sensitivity and speed. This is a significant achievement because it opens new possibilities for using THz technology in real-world applications—something that's been challenging to realize.'
The recent study by this team of researchers could have important implications for the development of various ultra-fast and highly performing THz technologies. For instance, it could inform the development of next-generation wireless communication technology (6G and beyond), navigation systems for autonomous vehicles and tools to capture high-resolution astronomical images.
'By improving our ability to detect THz light, we could also enhance industrial processes, like quality control and medical imaging, where this non-destructive sensing has shown promise,' said Bandurin.
'Looking ahead, our primary goal will be to refine these viscous electron bolometers and make them as effective and practical as possible for widespread use. We're exploring ways to optimize their sensitivity and durability, ensuring they can operate efficiently in a variety of conditions and applications.'
In their next studies, Bandurin and his colleagues also plan to study other quantum materials that exhibit similar fluid-like electron behaviors. This will allow them to determine if, compared to graphene, these materials exhibit similar or perhaps even more accentuated responses to THz radiation.
'Understanding how to harness this collective electron behavior more broadly could pave the way for even more advanced devices in optoelectronics and quantum technology, potentially leading to breakthroughs in data transmission, imaging, and beyond,' added Bandurin.
More information: M. Kravtsov et al, Viscous terahertz photoconductivity of hydrodynamic electrons in graphene, Nature Nanotechnology (2024). DOI: 10.1038/s41565-024-01795-y
© 2024 Science X Network