First Quantum Computer Network Demonstrated in Boston Metro Area
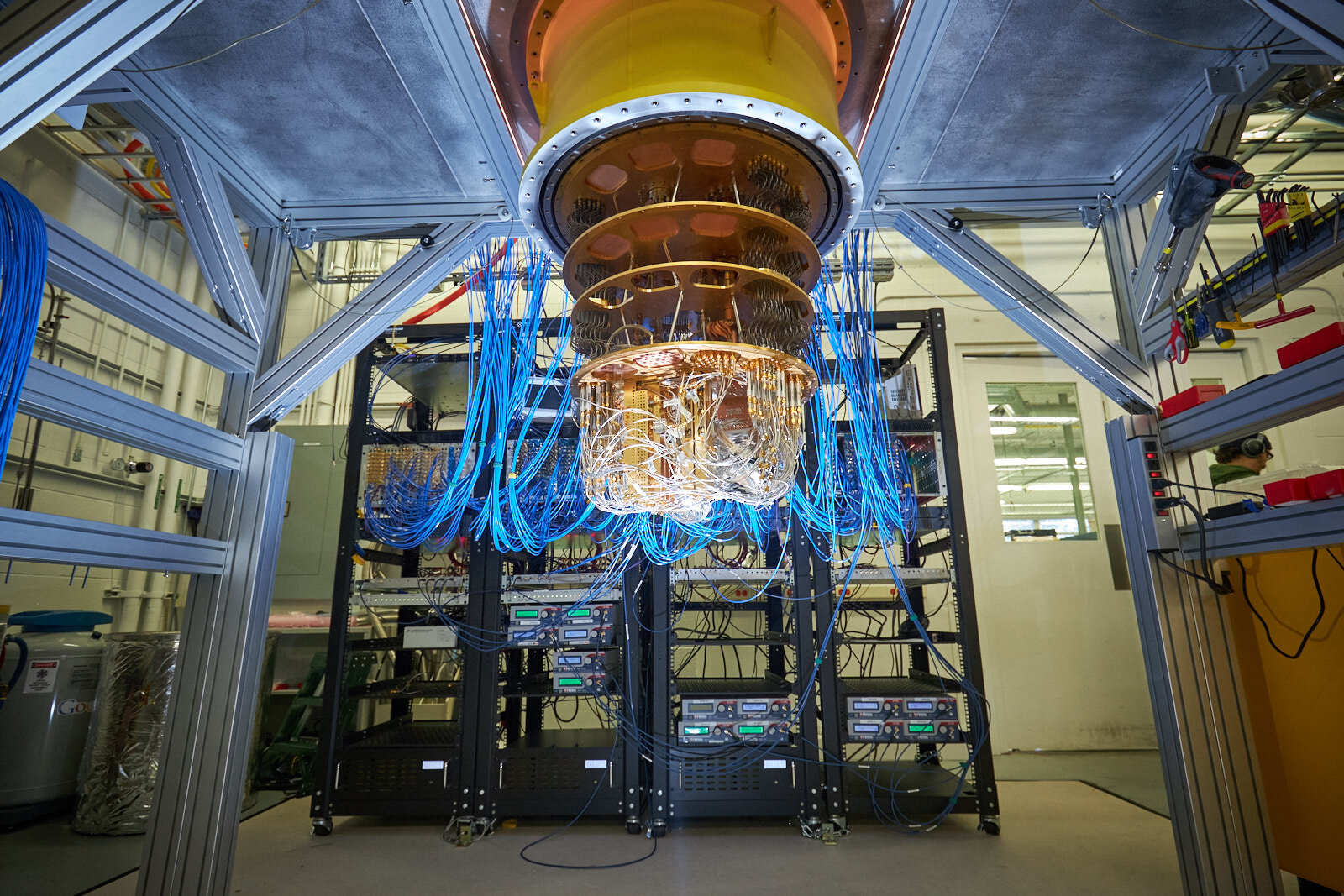
May 15, 2024
Following the stringent editorial process and policies of Science X, this article has been meticulously examined for its credibility. The editors have fact-checked the content, confirmed it as a peer-reviewed publication, validated the source, and proofread it. The review was performed by Harvard University.
The concept of a hacker-proof quantum internet that can transmit information globally with photons superimposed in diverse quantum states is nothing short of a dream. However, Harvard physicists have turned this dream into a concrete reality using the existing telecommunication fiber in the Boston area. They have presented a physical demonstration of the world's lengthiest fiber distance between two quantum memory nodes.
The spectacular breakthrough titled 'Entanglement of nanophotonic quantum memory nodes in a telecom network' was published in Nature. Mikhail Lukin, who led the work, collaborated with Harvard professors Marko Lončar and Hongkun Park, and researchers from Amazon Web Services, are part of the Harvard Quantum Initiative.
They have successfully designed the practical foundations of the inaugural quantum internet by entangling two quantum memory nodes separated by an optical fiber link covering nearly 22 miles loop through Cambridge, Somerville, Watertown, and Boston. The nodes were installed a floor apart in the Laboratory for Integrated Science and Engineering at Harvard.
Quantum memory, akin to classical computer memory, is the heart of a future interconnected quantum computing. It facilitates sophisticated network operations and stores and retrieves information. This longest fiber network created by the Harvard team outclasses past quantum networks in its potential to store, process, and relay data.
Each node, being a diminutive quantum computer, is crafted from a diamond shard with an atomic structural flaw termed a silicon-vacancy center. The interaction between silicon-vacancy centers and light escalates due to the carved structures inside the diamond, which are smaller than a hundredth of a human hair’s width.
The silicon-vacancy center houses two qubits (quantum information bits). One is in the form of an electron spin used for communication, and the other as a memory qubit to store entanglement. Both of these spins can be entirely controlled using microwave pulses. These few millimeters square diamond devices are housed inside dilution refrigeration units that can reach a temperature of -459°F.
The revolutionary technology employs silicon-vacancy centers as quantum memory devices for single photons. It resolves a major issue plaguing the theoretical quantum internet; the signal loss which cannot be amplified conventionally.
Copying arbitrary quantum information is impossible on a quantum network, making the information secure but hard to transport over great distances. However, silicon vacancy center-based network nodes can seize, store, and entangle quantum information bits while correcting signal loss. After chilling the nodes to nearly absolute zero, light is passed through the first node and becomes entangled with it.
As the photon is already entangled with the first node, this entanglement can be passed on to the second node, as explicated by the first author Can Knaut. This is referred to as photon-mediated entanglement.
The team has been running their experiments by leasing optical fiber from a Boston-based company over the past few years. They tested their demonstration network atop the existing fiber network, indicating the possibility of establishing a quantum internet with similar network lines.
Entangling quantum network nodes in a bustling urban environment signifies a noteworthy progression towards practical networking between quantum computers, as stated by Lukin. The research is far from over, with efforts ongoing to expand their network's performance by adding more nodes and experimenting with different networking protocols.
Journal information: Nature
Provided by Harvard University