Quantum Computing Breakthrough: Electron Charge Qubit Achieves Remarkable 0.1 Millisecond Coherence Time
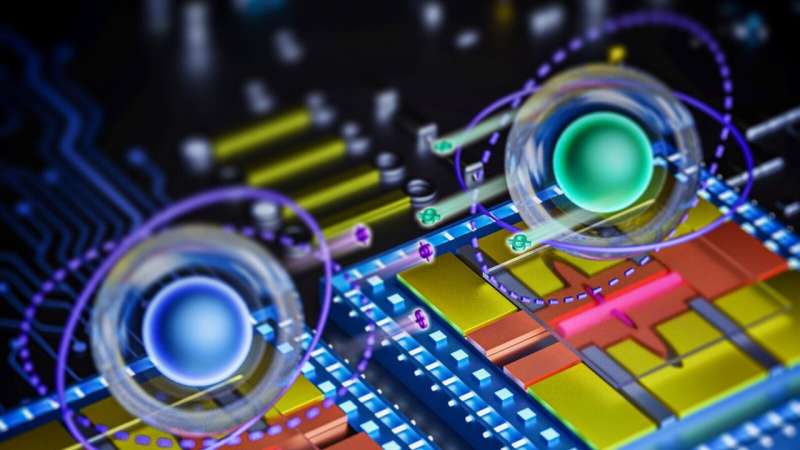
October 26, 2023
The article under discussion has been carefully reviewed following Science X's editorial practices and policies. Several parameters have been evaluated by Editors for verifying the credibility of the content:
- Fact-checking
- Peer-reviewed publication
- Source trustworthiness
- Proofreading
The review was conducted by Joseph E. Harmon from Argonne National Laboratory
The power of effective communication pivots on coherence, whether it is in terms of writing, speaking, or data processing. The concept is applicable even to quantum bits or qubits, which serve as fundamental units in quantum computing. A future quantum computer can address formidable tasks involving climate forecasting, design of materials, drug discovery, and more.
An important achievement has been made in the field of quantum computing by a team headed by the U.S. Department of Energy's (DOE) Argonne National Laboratory. The team has managed to increase the coherence time of a novel form of qubit to 0.1 milliseconds—a rate almost a thousand times higher than the last record. The achievement is described in a study published in Nature Physics.
Professor Dafei Jin from the University of Notre Dame who is also associated with Argonne's Center for Nanoscale Materials states "Our qubits can execute 10,000 operations with exceptional precision and speed, unlike conventional electron charge qubits that can perform just 10 to 100 over their coherence times".
In our day-to-day life, 0.1 milliseconds is an extremely brief time period. However, when it comes to the quantum realm, this duration enables a qubit to execute thousands of operations. Qubits, as compared to classical bits, have the potential to exist in both states, 0 and 1 concurrently.
Jin's team uses qubits that encode quantum data in the movement or charge states of an electron, hence they are termed as charge qubits. They have plenty of advantages such as ease of fabrication and operation, compatibility with traditional computing infrastructures, and low operational and construction costs for large-scale quantum computers.
Jin, while working in the Center for Nanoscale Materials (CNM), a DOE Office of Science user facility at Argonne, led the discovery of this novel type of qubit. The qubits are individual electrons captured on a solid and pristine neon surface in a vacuum. The resilience of neon in warding off disturbances arising from the surrounding environment and its non-reactivity with other elements makes it an ideal element. The neon environment offers protection to the electron qubit and inherently guarantees a lengthy coherence time.
The size of the qubits are minimal due to the small presence of single electrons on solid neon, making them compact and well-suited for up-scaling to multiple interconnected qubits.
In their pursuit to improve quantum computing, the team continued their experiments and not only elevated the quality of the neon surface but also managed to significantly bring down disruptive signals, resulting in a thousand-fold increase in coherence time from the original 0.1 microseconds.
In quantum computing, it is also crucial for a qubit to be scalable in order to link with numerous other qubits. The team successfully demonstrated that two-electron qubits can couple to the same superconducting circuit, thereby enabling the transfer of information between them through the circuit.
The team has not yet maximised the performance of their electron qubit. They have plans to further extend the coherence time and work on entangling two or more qubits.
In addition to Jin, Han and Li, Argonne contributors include postdocs Xianjing Zhou and Qianfan Chen. Other contributors include co-corresponding author David I. Schuster, a former physics professor at the University of Chicago now at Stanford University, and Xufeng Zhang, a former staff scientist at CNM and now a professor at Northeastern University. Also listed as authors are Gerwin Koolstra, Ge Yang, Brennan Dizdar, Yizhong Huang and Christopher S. Wang.
The collaborating institutions include Lawrence Berkeley National Laboratory, Massachusetts Institute of Technology, Northeastern University, Stanford University, the University of Chicago and the University of Notre Dame.
Journal information: Nature Physics
Provided by Argonne National Laboratory