New Window Unveiled to the Quantum World with Breakthrough Method
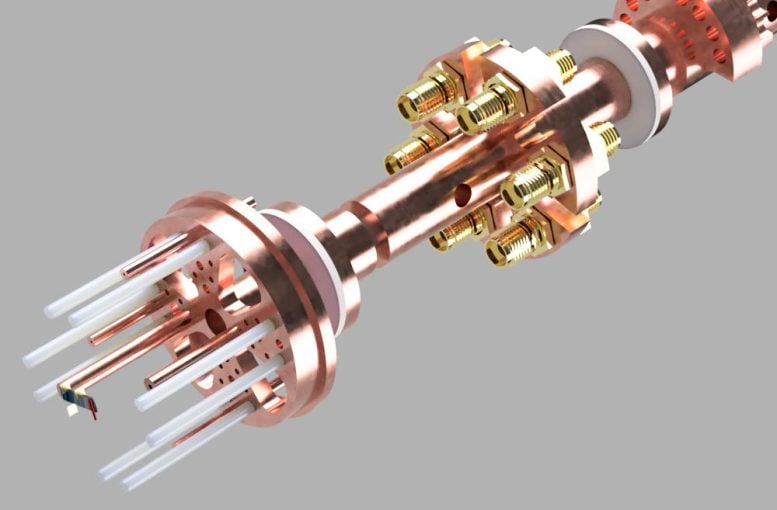
Groundbreaking developments in a new sample rod, featuring an advanced sample holder, offer the capacity for extremely precise temperature measurements. These advancements were spearheaded by D. Kojda/HZB
A team of scientists at HZB have unveiled a revolutionary approach to accurately detect tiny fluctuations in temperature, as small as 100 microkelvin, within the thermal Hall effect, addressing earlier restrictions posed by thermal interference. Through its application to terbium titanate, the researchers illustrated the power of this tool in producing reliable, reproducible results. Unraveling the thermal Hall effect provides insight into the behaviors of collective multi-particle states in quantum materials, especially their interactions with lattice vibrations or phonons.
All materials are governed by the laws of quantum physics, and quantum materials exhibit some rather unique properties as a result of these laws. For instance, temperature alterations or magnetic fields can stimulate excitations, collective states, or quasiparticles marked by phase transitions to distinct states. The understanding, management and control of these properties can enable their application in future information technologies that demands minimal energy consumption to handle or store data.
The Thermal Hall Effect (THE) is crucial in the identification of unique states in condensed matter. It is essentially founded on minute lateral temperature differences that appear when a thermal current is channeled through a sample and a perpendicular magnetic field is applied. Besides, the quantitative observation of the thermal Hall effect helps differentiate between unusual excitations and normal behavior.
The thermal Hall effect is apparent in a wide spectrum of materials including spin liquids, spin ice, high-temperature superconductors' parent phases, and materials with vastly polar properties. However, the extraordinarily small thermal differences which happen perpendicularly to the sample's temperature gradient have always posed a challenge to detect experimentally. Typically, in millimeter-sized samples, they range from microkelvins to millikelvins. The heat entry from measurement circuits and sensors usually veils the effect.
Pioneering work lead by PD Dr. Klaus Habicht, along with HZB sample environment specialists, has Jiu resulted in a revolutionary sample rod creation which can be inserted into a range of cryomagnets, due to its modular structure. The sample head measures the thermal Hall effect using capacitive thermometry, taking advantage of the temperature dependence of specially made miniature capacitors' capacitance. The team managed to notably minimize heat transfer via sensors and electronics, and decrease noise and interference signals. This was consolidated by analyzing a sample of terbium titanate.
Dr. Danny Kojda, the first author, shares his fascination with the capability of resolving temperature differences within the sub-millikelvin range, underlining it as key to furthering quantum materials study. He credits their success to the development of a refined experimental design accompanied by clear measurement protocols and precise analysis procedures which facilitate high accuracy and reproducible measurements. Department head, Klaus Habicht, expresses intent for further resolution improvement in future low sample temperature instruments, and his hope for this experimental setup integration into the HZB infrastructure with the intended upgrades introduced.
Habicht's group is now set upon using thermal Hall effect measurements to study the topological properties of phonons in quantum materials. Habicht expressed that the complete understanding of microscopic mechanisms and physics of scattering processes for the thermal Hall effect in ionic crystals remains elusive and it remains exciting to understand why electrically neutral quasiparticles in non-magnetic insulators are deflected in the magnetic field.