A Neutrino Mass Discrepancy Might Upend the Foundations of Cosmology
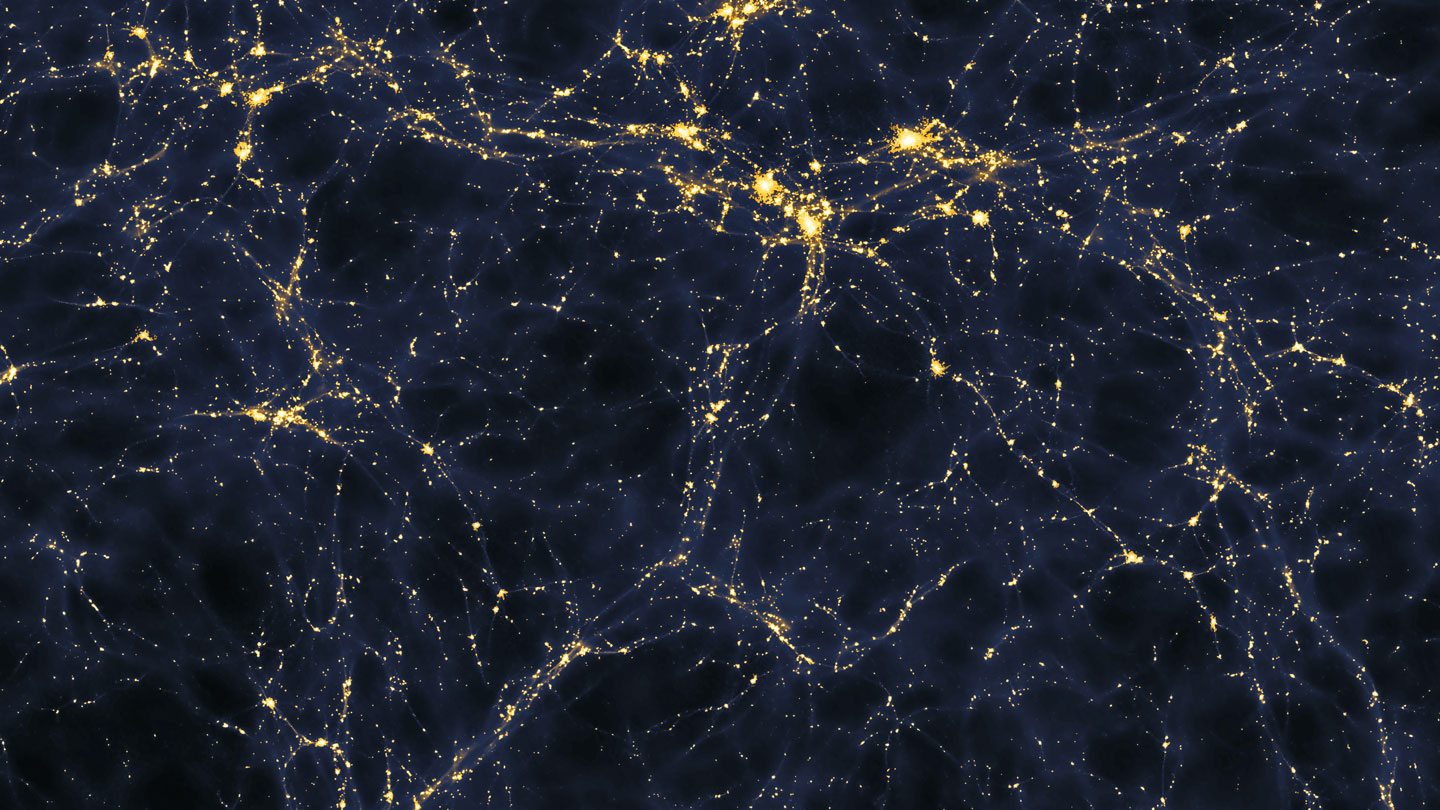
As the youthful universe congealed under the pull of gravity, matter knotted itself into galaxies, galaxy clusters and filaments, weaving a dazzlingly intricate cosmic web. This web’s structure is thanks, in part, to the handiwork of neutrinos — lightweight, subatomic particles that surge through the cosmos in unimaginable numbers.
Because they streak about at high speeds and rarely interact with other matter, the particles weren’t easily caught in the gravitational molasses of that latticework. So their presence swept away the cobwebs, hindering the formation of fine details in this cosmic filigree.
Science News is collecting reader questions about how to navigate our planet's changing climate.
What do you want to know about extreme heat and how it can lead to extreme weather events?
The masses of neutrinos are less than a millionth that of the next lightest particle, the electron, but no one knows exactly how massive they are. They are the only known type of fundamental subatomic particle for which this basic property is unknown, and some researchers suspect that this missing knowledge could be a gateway to a new understanding of physics.
“Neutrinos are one of the key particles that we do not understand as well as we do others, but that have nevertheless profound cosmological consequences,” says particle cosmologist Miguel Escudero of the European particle physics lab CERN near Geneva.
The little particles’ outsize role in sculpting the universe means they bridge the gap between the subatomic world, usually studied at particle accelerators or physics labs, and the cosmological one discerned by peering out at the heavens. So scientists are using both observations of space and experiments on the ground in an attempt to solve this massive mystery.
But if you ask a cosmologist how much neutrinos weigh and ask a particle physicist the same question, you might get two different answers. The two groups’ methods of gauging those masses are showing signs of a disconnect.
Recent cosmological data collected by the Dark Energy Spectroscopic Instrument, or DESI, favors masses that are unexpectedly small and creeping close to conflict with those of particle physics experiments. In fact, some interpretations of the DESI data suggest that neutrinos have no mass or even negative mass, normally a forbidden concept in physics (SN: 11/21/14).
The odd result has physicists considering some tantalizing ideas — that neutrinos’ masses might change over the history of the universe, or that the apparent negative masses are an illusion caused by dark energy, the mysterious phenomenon causing the universe to expand at an accelerating rate.
DESI, located at Kitt Peak National Observatory in Arizona, collects detailed maps of galaxies and other objects. In April, DESI scientists made a splash for suggesting that the density of dark energy might change over the history of the universe (SN: 4/4/24). The neutrino weirdness was overshadowed. But in the months since, physicists have realized that DESI might have big implications for neutrinos too.
Still, some scientists think the neutrino mass mismatch isn’t universe-shattering. Instead, it may result from abstruse details about how the cosmological data are analyzed.
But if the effect holds up, it could hint at a massive shift. “I think that our description of the universe is too simple,” says cosmologist Eleonora Di Valentino of the University of Sheffield in England. “Now that we have very strong and very sensitive measurements … it’s time to complicate it a bit.”
Neutrinos come in three varieties — electron neutrinos, muon neutrinos and tau neutrinos. To make matters more complicated, each type doesn’t have a definite mass but carries a quantum mixture of three different masses.
Today, the triumvirate suffuses the cosmos with hundreds of millions of neutrinos per cubic meter, outnumbering protons by a factor of about a billion. In the early universe, the particles were even more densely packed.
Although neutrinos are extremely lightweight, there’s strength in numbers. The particles have been throwing their weight around the cosmos for billions of years, indelibly etching the night sky with their presence. They flitted about not only the normal, visible matter that makes up stars and other spacefaring sundries, but also dark matter, a poorly understood source of mass that bulks up galaxies around the cosmos.
Neutrinos’ combined numbers were enough not only to alter the cosmic web, but also to influence the expansion rate of the universe. Those two factors allow scientists to gauge neutrino masses by peering into space. Neutrino masses on the bigger side would have resulted in a more rapid expansion of the universe and a less clumpy cosmos than smaller neutrino masses.
DESI maps out cosmic structures to determine that expansion rate, through an effect known as baryon acoustic oscillations, sound waves that imprinted circular patterns on the very early universe. By tracing those patterns at different points in the universe’s history, scientists can track its growth, a bit like cosmic tree rings.
Meanwhile, the cosmic microwave background, light released 380,000 years after the Big Bang, reveals the clumpiness of the cosmos. As light from the cosmic microwave background traverses space, its trajectory is bent by the pockets of matter on its journey, much like light passing through a lens. The amount of this gravitational lensing tells scientists how clumpy the cosmos is.
Combining the measurements of clumpiness from the cosmic microwave background and the expansion rate from DESI — two things that neutrinos affect — lets scientists zero in on their mass.
The DESI data, in combination with cosmic microwave background data from the European Space Agency’s Planck satellite, provide a mass ceiling for neutrinos. Specifically, the sum of the three neutrino masses is less than about 0.07 electron volts at 95 percent confidence level, researchers reported online in April at arXiv.org. (An electron volt is a unit physicists use to quantify mass. An electron’s mass is about 511,000 electron volts.)
In addition to a neutrino mass ceiling, there’s also a floor, based on laboratory particle physics experiments. Those experiments measure a phenomenon called neutrino oscillations, which results from the fact that each type of neutrino is a quantum mixture of different masses. The mass mélange means that neutrinos can change from one variety to another as they travel (SN: 10/6/15). What starts as a muon neutrino might later be detected as an electron neutrino.
Neutrino detectors can spot this shapeshifting. Because oscillations depend on the relationship between the different neutrino masses, these experiments can’t directly measure the masses themselves. But they do indicate that the sum of the three neutrino masses must be greater than about 0.06 electron volts.
That means DESI’s rejection of neutrino masses more than about 0.07 electron volts is disconcertingly close to ruling out the entire range of masses allowed by oscillation experiments. The floor and the ceiling are almost touching.
There’s still a little leeway — a crawl space, perhaps — for neutrino masses to live in harmony with both cosmology and oscillation experiments. But the DESI result is surprising for other reasons. For one, the value that DESI pinpoints as most likely for the sum of the neutrino masses is zero — no mass at all.
What’s more, when additional cosmological data are added to the DESI and Planck data, such as catalogs of exploding stars that also gauge the universe’s expansion rate, the upper limit on the mass shrinks further, to less than 0.05 electron volts, Di Valentino and colleagues reported July 25 at arXiv.org. The crawl space is essentially eliminated, leaving neutrino masses in a purgatory that’s difficult to explain without proposing new ideas about the cosmos.
“If you take everything at face value, which is a huge caveat…, then clearly we need new physics,” says cosmologist Sunny Vagnozzi of the University of Trento in Italy, another author of the paper
Even without the addition of the supernova data, the DESI result, if taken seriously, would answer a major question: Which neutrino is heaviest? The three neutrino masses are labeled rather uncreatively with the numbers 1, 2 and 3. In one possible scenario called the normal ordering, mass 3 is heavier than masses 1 and 2. In what’s known as the inverted ordering, masses 1 and 2 are heavier than 3. Another way of stating the problem: Are there two relatively light neutrino masses and one somewhat heavier one or two heavy and one light?
If the inverted ordering is correct, oscillation experiments imply the neutrino mass sum would be more than 0.1 electron volts. DESI squeezing the neutrino masses down to less than 0.07 electron volts not only leaves the normal ordering with little leeway, but it also seems to essentially rule out the inverted ordering.
“That’s why everybody’s going overboard,” says cosmologist Licia Verde of the University of Barcelona, a member of the DESI collaboration.
Nixing the inverted ordering would be a big deal, with repercussions for a slew of theories and experiments. The ordering is so important that scientists designed an enormous experiment — the Jiangmen Underground Neutrino Observatory in China, planned to start up this year — aimed at measuring it. But particle physicists are not canceling their plans, and no one is popping bottles of champagne to celebrate the demise of the inverted ordering.
The reason is that DESI’s mass ceiling exceeded expectations. “It was too good,” says cosmologist Daniel Green of the University of California, San Diego.
Given the amount of data DESI collected, scientists would have expected an upper limit that was more than twice as large, pegging the mass to less than about 0.18 electron volts, he says, leaving the possibility of the inverted ordering alive and well. In fact, DESI wasn’t expected to be able to rule out the inverted ordering — if the inverted ordering were incorrect — until it had taken several more years of data.
That has made physicists suspicious that something else is up.
If scientists take seriously DESI’s preference for zero neutrino mass, there are a few ways to explain it, despite the fact that neutrinos in the lab indisputably have mass. Neutrinos could decay into other particles or annihilate with one another, Green and colleagues suggest in a paper accepted in the Journal of High Energy Physics. Or perhaps neutrinos’ masses vary across time.
But there’s an even wilder possibility than zero mass: negative mass. Green suspected “all of this funny behavior was because the data was actually going the wrong way. [The data] was seeing the ‘opposite’ of a neutrino.” Namely, a neutrino with negative mass.
Whereas neutrinos with positive mass make the universe less clumpy, DESI and Planck might be finding the reverse, a universe that is clumpier than expected, meaning it has a larger-than-predicted variation in the density of matter from place to place. That could be conceptualized by a bizarro neutrino with negative mass.
In the DESI analysis, scientists didn’t allow the neutrino mass to go negative. Perhaps DESI landed on zero only because it was forbidden from going lower.
So Green and colleagues tweaked the analysis to permit negative masses. The analysis homed in on –0.16 electron volts, the researchers reported.
Others found similar support for negative neutrino masses. That’s “kind of a crazy thing to say,” says cosmologist Willem Elbers of Durham University in England. Negative masses in physics are hard to define and incorporate in theories, causing all kinds of conflict in equations. “We don’t actually think that the neutrino mass is negative,” Elbers says. Instead, “it’s a symptom of some problem either in the data or in the assumptions that we make about how the universe evolves.”
The negative mass could be a mirage of dark energy, Elbers and colleagues suggest. The standard picture of the universe assumes dark energy has a constant density, what’s known as a cosmological constant. While the DESI data hint that dark energy is dynamical — that its density changes over time — DESI’s neutrino mass number was determined assuming a cosmological constant.
Allowing dynamical dark energy resolves the neutrino mass issue, Elbers and colleagues reported online July 15 at arXiv.org. “It actually shifts the most likely value from something negative and unphysical to something that’s right on the mark,” Elbers says: 0.06 electron volts.
But not all dynamical dark energy is alike. The simplest models of dynamical dark energy, like that used by DESI and by Elbers and colleagues, allow dark energy to go “phantom,” an unexpected situation, theoretically. In scientists’ favorite theories, dark energy’s density either remains constant or gets diluted as space expands. With phantom dark energy, the density instead increases. That type of dark energy is considered less plausible — it’s difficult to explain within standard physics theories.
Using a model in which dark energy’s variation is prohibited from going phantom actually made the neutrino mass mismatch worse, Vagnozzi, Di Valentino and colleagues reported in their paper.
That leaves scientists with no winning cosmological explanation for why the neutrino masses are smaller than expected.
Rather than rethinking the universe, some scientists are taking a second look at the data.
Subtle issues in the cosmic microwave background data might be skewing things, some researchers suspect. In particular, the data from Planck is known to show an unexpected excess of gravitational lensing, that bending of the cosmic microwave background light that helps scientists deduce the neutrino masses.
More gravitational lensing is also what you’d expect from neutrinos with negative masses. In fact, earlier attempts to estimate the neutrino masses using Planck data combined with a predecessor of DESI also landed on unexpectedly small estimates. Perhaps Planck is the problem.
An updated version of the Planck data, using different methods of mapping out the cosmic microwave background, reduces this excessive gravitational lensing.
An analysis based on that updated Planck data, and removing two outlier DESI data points, eliminated the evidence for negative neutrino masses, Escudero and colleagues reported online July 18 at arXiv.org
Given that, Escudero says, “it seems premature to conclude there is a tension between the minimum value of neutrino masses we know from the laboratory and the lack of detection of neutrino masses in cosmology.”
But, he notes, the analysis still found no evidence of a positive mass for neutrinos.
The cosmological measurements of neutrino mass rely on a variety of observations, and they hinge on the correctness of scientists’ theory of the cosmos. If there’s a missing link anywhere, that makes the neutrino mass estimates unreliable. So in the future, scientists hope to measure the neutrino mass directly, on Earth.
The KATRIN experiment in Karlsruhe, Germany, searches for the influence of neutrinos masses on radioactive decays of tritium, a heavy form of hydrogen (SN: 4/21/21). When tritium’s nucleus decays, it emits an antineutrino (the antimatter twin of a neutrino) and an electron. KATRIN aims to detect the effect of antineutrinos’ masses on the energies of the electrons released in the decay.
But while experiments like this could theoretically measure neutrino mass, their results aren’t nearly as precise as those of cosmology. The sum of the neutrino masses must be less than 1.35 electron volts at 90 percent confidence level, KATRIN researchers reported online in June at arXiv.org. That’s a much weaker limit than cosmology puts on the mass. So even though direct experiments are considered more reliable, they’re not really telling scientists much that they didn’t already know. Future direct experiments may further zero in on neutrino mass, but if neutrino masses are as tiny as cosmologists think they are, it will take some serious technological advancements.
Still, the possibility of better understanding some of the most mysterious particles in the cosmos is tantalizing. “I find it particularly interesting that looking up at the sky can tell you something about a particle that is so light and tiny and small and subatomic,” Verde says.
And if scientists can find agreement between neutrinos on Earth and in space, they’ll have extra confidence that their theory of the universe is correct, Verde says. “If you can build a picture where everything hangs together, by combining both experiments that look at directly the infinitely small and experiments that look at the very big, it also offers support to the picture itself.”
Questions or comments on this article? E-mail us at feedback@sciencenews.org | Reprints FAQ
N. Craig et al. No νs is good news. Journal of High Energy Physics. In press, 2024.
J.-Q. Jiang et al. Neutrino cosmology after DESI: tightest mass upper limits, preference for the normal ordering, and tension with terrestrial observations. arXiv:2407.18047. Submitted July 25, 2024.
D. Naredo-Tuero et al. Living at the edge: a critical look at the cosmological neutrino mass bound. arXiv:2407.13831. Submitted Jul 18, 2024.
W. Elbers et al. Negative neutrino masses as a mirage of dark energy. arXiv:2407.10965. Submitted July 15, 2024.
DESI Collaboration. DESI 2024 VI: Cosmological constraints from the measurements of baryon acoustic oscillations. arXiv:2404.03002. Submitted April 3, 2024.
Physics writer Emily Conover has a Ph.D. in physics from the University of Chicago. She is a two-time winner of the D.C. Science Writers’ Association Newsbrief award.
We are at a critical time and supporting climate journalism is more important than ever. Science News and our parent organization, the Society for Science, need your help to strengthen environmental literacy and ensure that our response to climate change is informed by science.
Please subscribe to Science News and add $16 to expand science literacy and understanding.